Ionizable lipid nanoparticles encapsulating barcoded mRNA for accelerated in vivo delivery screening
- PMID: 31678653
- PMCID: PMC7032071
- DOI: 10.1016/j.jconrel.2019.10.028
Ionizable lipid nanoparticles encapsulating barcoded mRNA for accelerated in vivo delivery screening
Abstract
Messenger RNA (mRNA) has recently emerged as a promising class of nucleic acid therapy, with the potential to induce protein production to treat and prevent a range of diseases. However, the widespread use of mRNA as a therapeutic requires safe and effective in vivo delivery technologies. Libraries of ionizable lipid nanoparticles (LNPs) have been designed to encapsulate mRNA, prevent its degradation, and mediate intracellular delivery. However, these LNPs are typically characterized and screened in an in vitro setting, which may not fully replicate the biological barriers that they encounter in vivo. Here, we designed and evaluated a library of engineered LNPs containing barcoded mRNA (b-mRNA) to accelerate the screening of mRNA delivery platforms in vivo. These b-mRNA are similar in structure and function to regular mRNA, and contain barcodes that enable their delivery to be quantified via deep sequencing. Using a mini-library of b-mRNA LNPs formulated via microfluidic mixing, we show that these different formulations can be pooled together, administered intravenously into mice as a single pool, and their delivery to multiple organs (liver, spleen, brain, lung, heart, kidney, pancreas, and muscle) can be quantified simultaneously using deep sequencing. In the context of liver and spleen delivery, LNPs that exhibited high b-mRNA delivery also yielded high luciferase expression, indicating that this platform can identify lead LNP candidates as well as optimal formulation parameters for in vivo mRNA delivery. Interestingly, LNPs with identical formulation parameters that encapsulated different types of nucleic acid barcodes (b-mRNA versus a DNA barcode) altered in vivo delivery, suggesting that the structure of the barcoded nucleic acid affects LNP in vivo delivery. This platform, which enables direct barcoding and subsequent quantification of a functional mRNA, can accelerate the in vivo screening and design of LNPs for mRNA therapeutic applications such as CRISPR-Cas9 gene editing, mRNA vaccination, and other mRNA-based regenerative medicine and protein replacement therapies.
Keywords: Gene delivery; Gene therapy; High-throughput screening; Nanoparticle; mRNA.
Copyright © 2019 Elsevier B.V. All rights reserved.
Figures
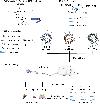
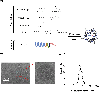
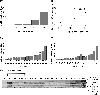
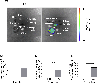
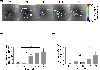
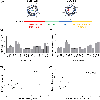
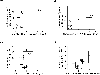
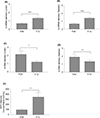
Similar articles
-
Helper lipid structure influences protein adsorption and delivery of lipid nanoparticles to spleen and liver.Biomater Sci. 2021 Feb 21;9(4):1449-1463. doi: 10.1039/d0bm01609h. Epub 2021 Jan 6. Biomater Sci. 2021. PMID: 33404020 Free PMC article.
-
Developing Biodegradable Lipid Nanoparticles for Intracellular mRNA Delivery and Genome Editing.Acc Chem Res. 2021 Nov 2;54(21):4001-4011. doi: 10.1021/acs.accounts.1c00500. Epub 2021 Oct 20. Acc Chem Res. 2021. PMID: 34668716 Review.
-
Lipid nanoparticle (LNP) mediated mRNA delivery in cardiovascular diseases: Advances in genome editing and CAR T cell therapy.J Control Release. 2024 Aug;372:113-140. doi: 10.1016/j.jconrel.2024.06.023. Epub 2024 Jun 15. J Control Release. 2024. PMID: 38876358 Review.
-
Testing the In Vitro and In Vivo Efficiency of mRNA-Lipid Nanoparticles Formulated by Microfluidic Mixing.J Vis Exp. 2023 Jan 20;(191). doi: 10.3791/64810. J Vis Exp. 2023. PMID: 36744791
-
Bile acid-containing lipid nanoparticles enhance extrahepatic mRNA delivery.Theranostics. 2024 Jan 1;14(1):1-16. doi: 10.7150/thno.89913. eCollection 2024. Theranostics. 2024. PMID: 38164140 Free PMC article.
Cited by
-
Ionizable drug delivery systems for efficient and selective gene therapy.Mil Med Res. 2023 Feb 27;10(1):9. doi: 10.1186/s40779-023-00445-z. Mil Med Res. 2023. PMID: 36843103 Free PMC article. Review.
-
Ionizable Lipid Nanoparticle-Mediated Delivery of Plasmid DNA in Cardiomyocytes.Int J Nanomedicine. 2022 Jun 30;17:2865-2881. doi: 10.2147/IJN.S366962. eCollection 2022. Int J Nanomedicine. 2022. PMID: 35795081 Free PMC article.
-
Comparison of the Immune Effects of an mRNA Vaccine and a Subunit Vaccine against Herpes Zoster Administered by Different Injection Methods.Vaccines (Basel). 2023 May 20;11(5):1003. doi: 10.3390/vaccines11051003. Vaccines (Basel). 2023. PMID: 37243107 Free PMC article.
-
Microfluidic Manufacture of Lipid-Based Nanomedicines.Pharmaceutics. 2022 Sep 14;14(9):1940. doi: 10.3390/pharmaceutics14091940. Pharmaceutics. 2022. PMID: 36145688 Free PMC article. Review.
-
Delivery of Plasmid DNA by Ionizable Lipid Nanoparticles to Induce CAR Expression in T Cells.Int J Nanomedicine. 2023 Oct 18;18:5891-5904. doi: 10.2147/IJN.S424723. eCollection 2023. Int J Nanomedicine. 2023. PMID: 37873551 Free PMC article.
References
-
- Cheng Q; Wei T; Jia Y; Farbiak L; Zhou K; Zhang S; Wei Y; Zhu H; Siegwart DJ, Dendrimer - Based Lipid Nanoparticles Deliver Therapeutic FAH mRNA to Normalize Liver Function and Extend Survival in a Mouse Model of Hepatorenal Tyrosinemia Type I. Advanced Materials 2018, 1805308. - PubMed
Publication types
MeSH terms
Substances
Grants and funding
LinkOut - more resources
Full Text Sources
Other Literature Sources