Branch site bulge conformations in domain 6 determine functional sugar puckers in group II intron splicing
- PMID: 31665419
- PMCID: PMC6868427
- DOI: 10.1093/nar/gkz965
Branch site bulge conformations in domain 6 determine functional sugar puckers in group II intron splicing
Abstract
Although group II intron ribozymes are intensively studied the question how structural dynamics affects splicing catalysis has remained elusive. We report for the first time that the group II intron domain 6 exists in a secondary structure equilibrium between a single- and a two-nucleotide bulge conformation, which is directly linked to a switch between sugar puckers of the branch site adenosine. Our study determined a functional sugar pucker equilibrium between the transesterification active C2'-endo conformation of the branch site adenosine in the 1nt bulge and an inactive C3'-endo state in the 2nt bulge fold, allowing the group II intron to switch its activity from the branching to the exon ligation step. Our detailed NMR spectroscopic investigation identified magnesium (II) ions and the branching reaction as regulators of the equilibrium populations. The tuneable secondary structure/sugar pucker equilibrium supports a conformational selection mechanism to up- and downregulate catalytically active and inactive states of the branch site adenosine to orchestrate the multi-step splicing process. The conformational dynamics of group II intron domain 6 is also proposed to be a key aspect for the directionality selection in reversible splicing.
© The Author(s) 2019. Published by Oxford University Press on behalf of Nucleic Acids Research.
Figures
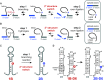
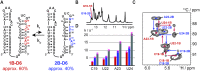
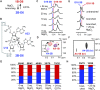
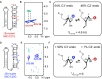
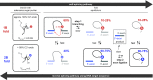
Similar articles
-
The receptor for branch-site docking within a group II intron active site.Mol Cell. 2006 Sep 15;23(6):831-40. doi: 10.1016/j.molcel.2006.07.017. Mol Cell. 2006. PMID: 16973435
-
More than one way to splice an RNA: branching without a bulge and splicing without branching in group II introns.RNA. 1998 Oct;4(10):1186-202. doi: 10.1017/s1355838298980724. RNA. 1998. PMID: 9769094 Free PMC article.
-
Unexpected metal ion requirements specific for catalysis of the branching reaction in a group II intron.Biochemistry. 1999 Mar 9;38(10):3157-67. doi: 10.1021/bi982462j. Biochemistry. 1999. PMID: 10074371
-
RNA splicing: group I intron crystal structures reveal the basis of splice site selection and metal ion catalysis.Curr Opin Struct Biol. 2006 Jun;16(3):319-26. doi: 10.1016/j.sbi.2006.04.005. Epub 2006 May 11. Curr Opin Struct Biol. 2006. PMID: 16697179 Review.
-
Group II intron lariat: Structural insights into the spliceosome.RNA Biol. 2015;12(9):913-7. doi: 10.1080/15476286.2015.1066956. RNA Biol. 2015. PMID: 26121424 Free PMC article. Review.
Cited by
-
Kinetic Resolution of the Atomic 3D Structures Formed by Ground and Excited Conformational States in an RNA Dynamic Ensemble.J Am Chem Soc. 2023 Oct 25;145(42):22964-22978. doi: 10.1021/jacs.3c04614. Epub 2023 Oct 13. J Am Chem Soc. 2023. PMID: 37831584 Free PMC article.
-
Structural basis for recognition of transcriptional terminator structures by ProQ/FinO domain RNA chaperones.Nat Commun. 2022 Nov 18;13(1):7076. doi: 10.1038/s41467-022-34875-5. Nat Commun. 2022. PMID: 36400772 Free PMC article.
-
The complete chloroplast genome of Photinia davidsoniae: molecular structures and comparative analysis.Mitochondrial DNA B Resour. 2021 Apr 19;6(4):1431-1439. doi: 10.1080/23802359.2021.1911698. Mitochondrial DNA B Resour. 2021. PMID: 33969192 Free PMC article.
-
Developments in solution-state NMR yield broader and deeper views of the dynamic ensembles of nucleic acids.Curr Opin Struct Biol. 2021 Oct;70:16-25. doi: 10.1016/j.sbi.2021.02.007. Epub 2021 Apr 6. Curr Opin Struct Biol. 2021. PMID: 33836446 Free PMC article. Review.
-
Comparative plastid genomics of four Pilea (Urticaceae) species: insight into interspecific plastid genome diversity in Pilea.BMC Plant Biol. 2021 Jan 7;21(1):25. doi: 10.1186/s12870-020-02793-7. BMC Plant Biol. 2021. PMID: 33413130 Free PMC article.
References
-
- Pyle A.M. Group II intron self-splicing. Annu. Rev. Biophys. 2016; 45:183–205. - PubMed
Publication types
MeSH terms
Substances
Grants and funding
LinkOut - more resources
Full Text Sources
Miscellaneous