Tel1 Activation by the MRX Complex Is Sufficient for Telomere Length Regulation but Not for the DNA Damage Response in Saccharomyces cerevisiae
- PMID: 31645360
- PMCID: PMC6893380
- DOI: 10.1534/genetics.119.302713
Tel1 Activation by the MRX Complex Is Sufficient for Telomere Length Regulation but Not for the DNA Damage Response in Saccharomyces cerevisiae
Abstract
Previous models suggested that regulation of telomere length in Saccharomyces cerevisiae by Tel1(ATM) and Mec1(ATR) would parallel the established pathways regulating the DNA damage response. Here, we provide evidence that telomere length regulation differs from the DNA damage response in both the Tel1 and Mec1 pathways. We found that Rad53 mediates a Mec1 telomere length regulation pathway but is dispensable for Tel1 telomere length regulation, whereas in the DNA damage response, Rad53 is regulated by both Mec1 and Tel1 Using epistasis analysis with a Tel1 hypermorphic allele, Tel1-hy909, we found that the MRX complex is not required downstream of Tel1 for telomere elongation but is required downstream of Tel1 for the DNA damage response. Our data suggest that nucleolytic telomere end processing is not a required step for telomerase to elongate telomeres.
Keywords: DNA damage response; MRX complex; Tel1; epistasis; telomere.
Copyright © 2019 by the Genetics Society of America.
Figures
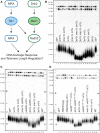
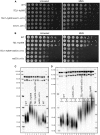
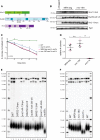
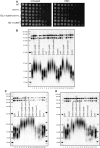
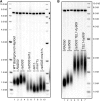
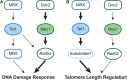
Similar articles
-
A balance between Tel1 and Rif2 activities regulates nucleolytic processing and elongation at telomeres.Mol Cell Biol. 2012 May;32(9):1604-17. doi: 10.1128/MCB.06547-11. Epub 2012 Feb 21. Mol Cell Biol. 2012. PMID: 22354991 Free PMC article.
-
Dominant TEL1-hy mutations compensate for Mec1 lack of functions in the DNA damage response.Mol Cell Biol. 2008 Jan;28(1):358-75. doi: 10.1128/MCB.01214-07. Epub 2007 Oct 22. Mol Cell Biol. 2008. PMID: 17954565 Free PMC article.
-
The telomere-binding protein Rif2 and ATP-bound Rad50 have opposing roles in the activation of yeast Tel1ATM kinase.J Biol Chem. 2019 Dec 6;294(49):18846-18852. doi: 10.1074/jbc.RA119.011077. Epub 2019 Oct 22. J Biol Chem. 2019. PMID: 31640985 Free PMC article.
-
Interplays between ATM/Tel1 and ATR/Mec1 in sensing and signaling DNA double-strand breaks.DNA Repair (Amst). 2013 Oct;12(10):791-9. doi: 10.1016/j.dnarep.2013.07.009. Epub 2013 Aug 13. DNA Repair (Amst). 2013. PMID: 23953933 Review.
-
Budding yeast Rad51: a paradigm for how phosphorylation and intrinsic structural disorder regulate homologous recombination and protein homeostasis.Curr Genet. 2021 Jun;67(3):389-396. doi: 10.1007/s00294-020-01151-2. Epub 2021 Jan 12. Curr Genet. 2021. PMID: 33433732 Free PMC article. Review.
Cited by
-
Rif2 interaction with Rad50 counteracts Tel1 functions in checkpoint signalling and DNA tethering by releasing Tel1 from MRX binding.Nucleic Acids Res. 2024 Mar 21;52(5):2355-2371. doi: 10.1093/nar/gkad1246. Nucleic Acids Res. 2024. PMID: 38180815 Free PMC article.
-
Mre11-Rad50 oligomerization promotes DNA double-strand break repair.Nat Commun. 2022 May 2;13(1):2374. doi: 10.1038/s41467-022-29841-0. Nat Commun. 2022. PMID: 35501303 Free PMC article.
-
Suppression of cdc13-2-associated senescence by pif1-m2 requires Ku-mediated telomerase recruitment.G3 (Bethesda). 2022 Jan 4;12(1):jkab360. doi: 10.1093/g3journal/jkab360. G3 (Bethesda). 2022. PMID: 34751785 Free PMC article.
-
Mechanism of MRX inhibition by Rif2 at telomeres.Nat Commun. 2021 May 12;12(1):2763. doi: 10.1038/s41467-021-23035-w. Nat Commun. 2021. PMID: 33980827 Free PMC article.
-
Profiling Tel1 Signaling Reveals a Non-Canonical Motif Targeting DNA Repair and Telomere Control Machineries.bioRxiv [Preprint]. 2024 Jul 5:2024.07.03.601872. doi: 10.1101/2024.07.03.601872. bioRxiv. 2024. PMID: 39005478 Free PMC article. Preprint.
References
-
- Anand R., Memisoglu G. and Haber J., 2017. Cas9-mediated gene editing in Saccharomyces cerevisiae. Protoc. Exch. 10.1038/protex.2017.021a - DOI
Publication types
MeSH terms
Substances
Grants and funding
LinkOut - more resources
Full Text Sources
Molecular Biology Databases
Research Materials
Miscellaneous