Characterization and mutagenesis of Chinese hamster ovary cells endogenous retroviruses to inactivate viral particle release
- PMID: 31631325
- PMCID: PMC7003738
- DOI: 10.1002/bit.27200
Characterization and mutagenesis of Chinese hamster ovary cells endogenous retroviruses to inactivate viral particle release
Abstract
The Chinese hamster ovary (CHO) cells used to produce biopharmaceutical proteins are known to contain type-C endogenous retrovirus (ERV) sequences in their genome and to release retroviral-like particles. Although evidence for their infectivity is missing, this has raised safety concerns. As the genomic origin of these particles remained unclear, we characterized type-C ERV elements at the genome, transcriptome, and viral particle RNA levels. We identified 173 type-C ERV sequences clustering into three functionally conserved groups. Transcripts from one type-C ERV group were full-length, with intact open reading frames, and cognate viral genome RNA was loaded into retroviral-like particles, suggesting that this ERV group may produce functional viruses. CRISPR-Cas9 genome editing was used to disrupt the gag gene of the expressed type-C ERV group. Comparison of CRISPR-derived mutations at the DNA and RNA level led to the identification of a single ERV as the main source of the release of RNA-loaded viral particles. Clones bearing a Gag loss-of-function mutation in this ERV showed a reduction of RNA-containing viral particle release down to detection limits, without compromising cell growth or therapeutic protein production. Overall, our study provides a strategy to mitigate potential viral particle contaminations resulting from ERVs during biopharmaceutical manufacturing.
Keywords: Chinese hamster ovary cells; adventitious agents; endogenous retroviral elements; genome editing.
© 2019 The Authors. Biotechnology and Bioengineering published by Wiley Periodicals, Inc.
Conflict of interest statement
Some of the authors are employed by and/or are consultants of Selexis SA, a company generating therapeutic protein‐producing CHO cell lines. P.O.D. and S.B. university positions were funded in part by the Swiss government, the University of Lausanne and Selexis SA.
Figures
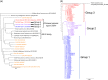
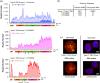
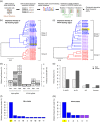
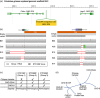
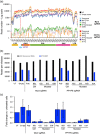
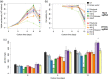
Similar articles
-
Defective endogenous retrovirus-like sequences and particles of Chinese hamster ovary cells.Dev Biol Stand. 1991;75:123-32. Dev Biol Stand. 1991. PMID: 1665459
-
Identification and spontaneous immune targeting of an endogenous retrovirus K envelope protein in the Indian rhesus macaque model of human disease.Retrovirology. 2016 Jan 15;13:6. doi: 10.1186/s12977-016-0238-0. Retrovirology. 2016. PMID: 26767784 Free PMC article.
-
New bioinformatic tool for quick identification of functionally relevant endogenous retroviral inserts in human genome.Cell Cycle. 2015;14(9):1476-84. doi: 10.1080/15384101.2015.1022696. Cell Cycle. 2015. PMID: 25853282 Free PMC article.
-
Tracking the Continuous Evolutionary Processes of an Endogenous Retrovirus of the Domestic Cat: ERV-DC.Viruses. 2018 Apr 6;10(4):179. doi: 10.3390/v10040179. Viruses. 2018. PMID: 29642384 Free PMC article. Review.
-
The role of human endogenous retroviruses in melanoma.Br J Dermatol. 2009 Dec;161(6):1225-31. doi: 10.1111/j.1365-2133.2009.09415.x. Epub 2009 Jul 20. Br J Dermatol. 2009. PMID: 19785608 Review.
Cited by
-
Preclinical evaluation of manufacturable SARS-CoV-2 spike virus-like particles produced in Chinese Hamster Ovary cells.Commun Med (Lond). 2023 Aug 23;3(1):116. doi: 10.1038/s43856-023-00340-7. Commun Med (Lond). 2023. PMID: 37612423 Free PMC article.
-
HervD Atlas: a curated knowledgebase of associations between human endogenous retroviruses and diseases.Nucleic Acids Res. 2024 Jan 5;52(D1):D1315-D1326. doi: 10.1093/nar/gkad904. Nucleic Acids Res. 2024. PMID: 37870452 Free PMC article.
-
Multiplex secretome engineering enhances recombinant protein production and purity.Nat Commun. 2020 Apr 20;11(1):1908. doi: 10.1038/s41467-020-15866-w. Nat Commun. 2020. PMID: 32313013 Free PMC article.
-
CRISPR Technologies in Chinese Hamster Ovary Cell Line Engineering.Int J Mol Sci. 2023 May 2;24(9):8144. doi: 10.3390/ijms24098144. Int J Mol Sci. 2023. PMID: 37175850 Free PMC article. Review.
-
Cell culture bioprocessing - the road taken and the path forward.Curr Opin Chem Eng. 2020 Dec;30:100663. doi: 10.1016/j.coche.2020.100663. Epub 2020 Dec 1. Curr Opin Chem Eng. 2020. PMID: 33391982 Free PMC article.
References
-
- Aguirre, A. J. , Meyers, R. M. , Weir, B. A. , Vazquez, F. , Zhang, C. Z. , Ben‐David, U. , & Hahn, W. C. (2016). Genomic copy number dictates a gene‐independent cell response to CRISPR/Cas9 targeting. Cancer Discovery, 6(8), 914–929. 10.1158/2159-8290.CD-16-0154 - DOI - PMC - PubMed
Publication types
MeSH terms
Substances
Grants and funding
LinkOut - more resources
Full Text Sources
Other Literature Sources
Research Materials