Differential Modulation of Innate Immune Responses in Human Primary Cells by Influenza A Viruses Carrying Human or Avian Nonstructural Protein 1
- PMID: 31597767
- PMCID: PMC6912104
- DOI: 10.1128/JVI.00999-19
Differential Modulation of Innate Immune Responses in Human Primary Cells by Influenza A Viruses Carrying Human or Avian Nonstructural Protein 1
Abstract
The influenza A virus (IAV) nonstructural protein 1 (NS1) contributes to disease pathogenesis through the inhibition of host innate immune responses. Dendritic cells (DCs) release interferons (IFNs) and proinflammatory cytokines and promote adaptive immunity upon viral infection. In order to characterize the strain-specific effects of IAV NS1 on human DC activation, we infected human DCs with a panel of recombinant viruses with the same backbone (A/Puerto Rico/08/1934) expressing different NS1 proteins from human and avian origin. We found that these viruses induced a clearly distinct phenotype in DCs. Specifically, viruses expressing NS1 from human IAV (either H1N1 or H3N2) induced higher levels of expression of type I (IFN-α and IFN-β) and type III (IFN-λ1 to IFNλ3) IFNs than viruses expressing avian IAV NS1 proteins (H5N1, H7N9, and H7N2), but the differences observed in the expression levels of proinflammatory cytokines like tumor necrosis factor alpha (TNF-α) or interleukin-6 (IL-6) were not significant. In addition, using imaging flow cytometry, we found that human and avian NS1 proteins segregate based on their subcellular trafficking dynamics, which might be associated with the different innate immune profile induced in DCs by viruses expressing those NS1 proteins. Innate immune responses induced by our panel of IAV recombinant viruses were also characterized in normal human bronchial epithelial cells, and the results were consistent with those in DCs. Altogether, our results reveal an increased ability of NS1 from avian viruses to antagonize innate immune responses in human primary cells compared to the ability of NS1 from human viruses, which could contribute to the severe disease induced by avian IAV in humans.IMPORTANCE Influenza A viruses (IAVs) cause seasonal epidemics which result in an important health and economic burden. Wild aquatic birds are the natural host of IAV. However, IAV can infect diverse hosts, including humans, domestic poultry, pigs, and others. IAVs circulating in animals occasionally cross the species barrier, infecting humans, which results in mild to very severe disease. In some cases, these viruses can acquire the ability to be transmitted among humans and initiate a pandemic. The nonstructural 1 (NS1) protein of IAV is an important antagonist of the innate immune response. In this study, using recombinant viruses and primary human cells, we show that NS1 proteins from human and avian hosts show intrinsic differences in the modulation of the innate immunity in human dendritic cells and epithelial cells, as well as different cellular localization dynamics in infected cells.
Keywords: NS1 protein; dendritic cells; epithelial cells; influenza; innate immunity; interferons.
Copyright © 2019 Monteagudo et al.
Figures
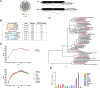
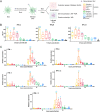
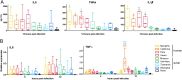
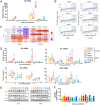
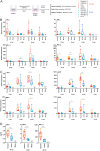
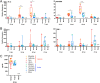
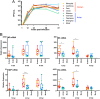
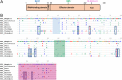
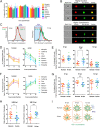
Similar articles
-
Mammalian Adaptation of an Avian Influenza A Virus Involves Stepwise Changes in NS1.J Virol. 2018 Feb 12;92(5):e01875-17. doi: 10.1128/JVI.01875-17. Print 2018 Mar 1. J Virol. 2018. PMID: 29237841 Free PMC article.
-
A Novel Role for PDZ-Binding Motif of Influenza A Virus Nonstructural Protein 1 in Regulation of Host Susceptibility to Postinfluenza Bacterial Superinfections.Viral Immunol. 2019 Apr;32(3):131-143. doi: 10.1089/vim.2018.0118. Epub 2019 Mar 1. Viral Immunol. 2019. PMID: 30822217 Free PMC article.
-
pH Optimum of Hemagglutinin-Mediated Membrane Fusion Determines Sensitivity of Influenza A Viruses to the Interferon-Induced Antiviral State and IFITMs.J Virol. 2017 May 12;91(11):e00246-17. doi: 10.1128/JVI.00246-17. Print 2017 Jun 1. J Virol. 2017. PMID: 28356532 Free PMC article.
-
Modulation of Innate Immune Responses by the Influenza A NS1 and PA-X Proteins.Viruses. 2018 Dec 12;10(12):708. doi: 10.3390/v10120708. Viruses. 2018. PMID: 30545063 Free PMC article. Review.
-
Innate immune responses to influenza A H5N1: friend or foe?Trends Immunol. 2009 Dec;30(12):574-84. doi: 10.1016/j.it.2009.09.004. Epub 2009 Oct 26. Trends Immunol. 2009. PMID: 19864182 Free PMC article. Review.
Cited by
-
Functional Characterization and Direct Comparison of Influenza A, B, C, and D NS1 Proteins in vitro and in vivo.Front Microbiol. 2019 Dec 17;10:2862. doi: 10.3389/fmicb.2019.02862. eCollection 2019. Front Microbiol. 2019. PMID: 31921042 Free PMC article.
-
Antiviral responses versus virus-induced cellular shutoff: a game of thrones between influenza A virus NS1 and SARS-CoV-2 Nsp1.Front Cell Infect Microbiol. 2024 Feb 5;14:1357866. doi: 10.3389/fcimb.2024.1357866. eCollection 2024. Front Cell Infect Microbiol. 2024. PMID: 38375361 Free PMC article. Review.
-
Recombinant Influenza A Viruses Expressing Reporter Genes from the Viral NS Segment.Int J Mol Sci. 2024 Oct 1;25(19):10584. doi: 10.3390/ijms251910584. Int J Mol Sci. 2024. PMID: 39408912 Free PMC article. Review.
-
Human genes with codon usage bias similar to that of the nonstructural protein 1 gene of influenza A viruses are conjointly involved in the infectious pathogenesis of influenza A viruses.Genetica. 2022 Apr;150(2):97-115. doi: 10.1007/s10709-022-00155-9. Epub 2022 Apr 8. Genetica. 2022. PMID: 35396627 Free PMC article.
-
Influenza A virus NS1 optimises virus infectivity by enhancing genome packaging in a dsRNA-binding dependent manner.Virol J. 2020 Jul 16;17(1):107. doi: 10.1186/s12985-020-01357-3. Virol J. 2020. PMID: 32677963 Free PMC article.
References
Publication types
MeSH terms
Substances
Grants and funding
LinkOut - more resources
Full Text Sources
Medical
Research Materials