Astroglia in Alzheimer's Disease
- PMID: 31583592
- PMCID: PMC7266005
- DOI: 10.1007/978-981-13-9913-8_11
Astroglia in Alzheimer's Disease
Abstract
Alzheimer's disease is the most common cause of dementia. Cellular changes in the brains of the patients suffering from Alzheimer's disease occur well in advance of the clinical symptoms. At the cellular level, the most dramatic is a demise of neurones. As astroglial cells carry out homeostatic functions of the brain, it is certain that these cells are at least in part a cause of Alzheimer's disease. Historically, Alois Alzheimer himself has recognised this at the dawn of the disease description. However, the role of astroglia in this disease has been understudied. In this chapter, we summarise the various aspects of glial contribution to this disease and outline the potential of using these cells in prevention (exercise and environmental enrichment) and intervention of this devastating disease.
Keywords: Alzheimer’s disease; Astrocytes; Astroglial atrophy; Calcium signalling; Neurodegeneration; Pathological ageing; Stem cells.
Figures
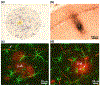
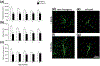
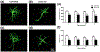
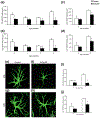
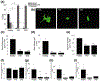
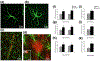
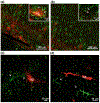
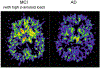
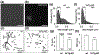
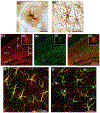
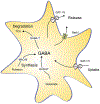
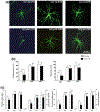
Similar articles
-
Astroglia in dementia and Alzheimer's disease.Cell Death Differ. 2009 Mar;16(3):378-85. doi: 10.1038/cdd.2008.172. Epub 2008 Dec 5. Cell Death Differ. 2009. PMID: 19057621 Review.
-
Astroglial atrophy in Alzheimer's disease.Pflugers Arch. 2019 Oct;471(10):1247-1261. doi: 10.1007/s00424-019-02310-2. Epub 2019 Sep 13. Pflugers Arch. 2019. PMID: 31520182 Review.
-
Astrocytes in physiological aging and Alzheimer's disease.Neuroscience. 2016 May 26;323:170-82. doi: 10.1016/j.neuroscience.2015.01.007. Epub 2015 Jan 14. Neuroscience. 2016. PMID: 25595973 Review.
-
Regressive changes of astroglia in white matter lesions in cerebrovascular disease and Alzheimer's disease patients.Acta Neuropathol. 1997 Aug;94(2):146-52. doi: 10.1007/s004010050686. Acta Neuropathol. 1997. PMID: 9255389
-
The role of astroglia in Alzheimer's disease: pathophysiology and clinical implications.Lancet Neurol. 2019 Apr;18(4):406-414. doi: 10.1016/S1474-4422(18)30490-3. Epub 2019 Feb 19. Lancet Neurol. 2019. PMID: 30795987 Review.
Cited by
-
TGF-β as a Key Modulator of Astrocyte Reactivity: Disease Relevance and Therapeutic Implications.Biomedicines. 2022 May 23;10(5):1206. doi: 10.3390/biomedicines10051206. Biomedicines. 2022. PMID: 35625943 Free PMC article. Review.
-
Overdosing on iron: Elevated iron and degenerative brain disorders.Exp Biol Med (Maywood). 2020 Oct;245(16):1444-1473. doi: 10.1177/1535370220953065. Epub 2020 Sep 2. Exp Biol Med (Maywood). 2020. PMID: 32878460 Free PMC article. Review.
-
High-Throughput Analysis of Astrocyte Cultures Shows Prevention of Reactive Astrogliosis by the Multi-Nutrient Combination Fortasyn Connect.Cells. 2022 Apr 22;11(9):1428. doi: 10.3390/cells11091428. Cells. 2022. PMID: 35563732 Free PMC article.
-
Gene Enrichment Analysis of Astrocyte Subtypes in Psychiatric Disorders and Psychotropic Medication Datasets.Cells. 2022 Oct 21;11(20):3315. doi: 10.3390/cells11203315. Cells. 2022. PMID: 36291180 Free PMC article.
-
R-Ras GTPases Signaling Role in Myelin Neurodegenerative Diseases.Int J Mol Sci. 2020 Aug 17;21(16):5911. doi: 10.3390/ijms21165911. Int J Mol Sci. 2020. PMID: 32824627 Free PMC article. Review.
References
-
- Alberdi E, Wyssenbach A, Alberdi M, Sanchez-Gomez MV, Cavaliere F, Rodriguez JJ, Verkhratsky A, Matute C (2013) Ca2+-dependent endoplasmic reticulum stress correlates with astrogliosis in oligomeric amyloid β-treated astrocytes and in a model of Alzheimer’s disease. Aging Cell 12:292–302 - PubMed
Publication types
MeSH terms
Grants and funding
LinkOut - more resources
Full Text Sources
Medical
Research Materials