Broad Cross-Species Infection of Cultured Cells by Bat HKU2-Related Swine Acute Diarrhea Syndrome Coronavirus and Identification of Its Replication in Murine Dendritic Cells In Vivo Highlight Its Potential for Diverse Interspecies Transmission
- PMID: 31554686
- PMCID: PMC6880172
- DOI: 10.1128/JVI.01448-19
Broad Cross-Species Infection of Cultured Cells by Bat HKU2-Related Swine Acute Diarrhea Syndrome Coronavirus and Identification of Its Replication in Murine Dendritic Cells In Vivo Highlight Its Potential for Diverse Interspecies Transmission
Abstract
Outbreaks of severe diarrhea in neonatal piglets in Guangdong, China, in 2017 resulted in the isolation and discovery of a novel swine enteric alphacoronavirus (SeACoV) derived from the species Rhinolophus bat coronavirus HKU2 (Y. Pan, X. Tian, P. Qin, B. Wang, et al., Vet Microbiol 211:15-21, 2017). SeACoV was later referred to as swine acute diarrhea syndrome CoV (SADS-CoV) by another group (P. Zhou, H. Fan, T. Lan, X.-L. Yang, et al., Nature 556:255-258, 2018). The present study was set up to investigate the potential species barriers of SADS-CoV in vitro and in vivo We first demonstrated that SADS-CoV possesses a broad species tropism and is able to infect cell lines from diverse species, including bats, mice, rats, gerbils, hamsters, pigs, chickens, nonhuman primates, and humans. Trypsin contributes to but is not essential for SADS-CoV propagation in vitro Furthermore, C57BL/6J mice were inoculated with the virus via oral or intraperitoneal routes. Although the mice exhibited only subclinical infection, they supported viral replication and prolonged infection in the spleen. SADS-CoV nonstructural proteins and double-stranded RNA were detected in splenocytes of the marginal zone on the edge of lymphatic follicles, indicating active replication of SADS-CoV in the mouse model. We identified that splenic dendritic cells (DCs) are the major targets of virus infection by immunofluorescence and flow cytometry approaches. Finally, we demonstrated that SADS-CoV does not utilize known CoV receptors for cellular entry. The ability of SADS-CoV to replicate in various cells lines from a broad range of species and the unexpected tropism for murine DCs provide important insights into the biology of this bat-origin CoV, highlighting its possible ability to cross interspecies barriers.IMPORTANCE Infections with bat-origin coronaviruses (CoVs) (severe acute respiratory syndrome CoV [SARS-CoV] and Middle East respiratory syndrome CoV [MERS-CoV]) have caused severe illness in humans after "host jump" events. Recently, a novel bat-HKU2-like CoV named swine acute diarrhea syndrome CoV (SADS-CoV) has emerged in southern China, causing lethal diarrhea in newborn piglets. It is important to assess the species barriers of SADS-CoV infection since the animal hosts (other than pigs and bats) and zoonotic potential are still unknown. An in vitro susceptibility study revealed a broad species tropism of SADS-CoV, including various rodent and human cell lines. We established a mouse model of SADS-CoV infection, identifying its active replication in splenic dendritic cells, which suggests that SADS-CoV has the potential to infect rodents. These findings highlight the potential cross-species transmissibility of SADS-CoV, although further surveillance in other animal populations is needed to fully understand the ecology of this bat-HKU2-origin CoV.
Keywords: Coronavirus; SADS-CoV; interspecies transmission; mouse infection model.
Copyright © 2019 American Society for Microbiology.
Figures
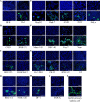
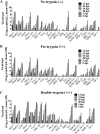
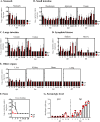
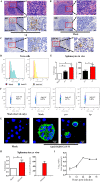
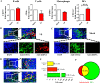
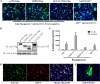
Comment in
-
Broad Cell Tropism of SADS-CoV In Vitro Implies Its Potential Cross-Species Infection Risk.Virol Sin. 2021 Jun;36(3):559-563. doi: 10.1007/s12250-020-00321-3. Epub 2020 Nov 17. Virol Sin. 2021. PMID: 33201410 Free PMC article. No abstract available.
Similar articles
-
Identification of ZDHHC17 as a Potential Drug Target for Swine Acute Diarrhea Syndrome Coronavirus Infection.mBio. 2021 Oct 26;12(5):e0234221. doi: 10.1128/mBio.02342-21. Epub 2021 Oct 26. mBio. 2021. PMID: 34700373 Free PMC article.
-
Interspecies Jumping of Bat Coronaviruses.Viruses. 2021 Oct 29;13(11):2188. doi: 10.3390/v13112188. Viruses. 2021. PMID: 34834994 Free PMC article. Review.
-
Fatal swine acute diarrhoea syndrome caused by an HKU2-related coronavirus of bat origin.Nature. 2018 Apr;556(7700):255-258. doi: 10.1038/s41586-018-0010-9. Epub 2018 Apr 4. Nature. 2018. PMID: 29618817 Free PMC article.
-
Bat-Origin Swine Acute Diarrhea Syndrome Coronavirus Is Lethal to Neonatal Mice.J Virol. 2023 Mar 30;97(3):e0019023. doi: 10.1128/jvi.00190-23. Epub 2023 Mar 6. J Virol. 2023. PMID: 36877051 Free PMC article.
-
Swine enteric alphacoronavirus (swine acute diarrhea syndrome coronavirus): An update three years after its discovery.Virus Res. 2020 Aug;285:198024. doi: 10.1016/j.virusres.2020.198024. Epub 2020 May 16. Virus Res. 2020. PMID: 32482591 Free PMC article. Review.
Cited by
-
Getting Insights from a Large Corpus of Scientific Papers on Specialisted Comprehensive Topics - the Case of COVID-19.Procedia Comput Sci. 2020;176:2287-2296. doi: 10.1016/j.procs.2020.09.287. Epub 2020 Oct 2. Procedia Comput Sci. 2020. PMID: 33042309 Free PMC article.
-
Swine acute diarrhea syndrome coronavirus replication in primary human cells reveals potential susceptibility to infection.Proc Natl Acad Sci U S A. 2020 Oct 27;117(43):26915-26925. doi: 10.1073/pnas.2001046117. Epub 2020 Oct 12. Proc Natl Acad Sci U S A. 2020. PMID: 33046644 Free PMC article.
-
Coronavirus Infection and Cholesterol Metabolism.Front Immunol. 2022 Apr 21;13:791267. doi: 10.3389/fimmu.2022.791267. eCollection 2022. Front Immunol. 2022. PMID: 35529872 Free PMC article. Review.
-
Development and Clinical Applications of a 5-Plex Real-Time RT-PCR for Swine Enteric Coronaviruses.Viruses. 2022 Jul 14;14(7):1536. doi: 10.3390/v14071536. Viruses. 2022. PMID: 35891517 Free PMC article.
-
Roles of Two Major Domains of the Porcine Deltacoronavirus S1 Subunit in Receptor Binding and Neutralization.J Virol. 2021 Nov 23;95(24):e0111821. doi: 10.1128/JVI.01118-21. Epub 2021 Sep 22. J Virol. 2021. PMID: 34549985 Free PMC article.
References
-
- van Boheemen S, de Graaf M, Lauber C, Bestebroer TM, Raj VS, Zaki AM, Osterhaus AD, Haagmans BL, Gorbalenya AE, Snijder EJ, Fouchier RA. 2012. Genomic characterization of a newly discovered coronavirus associated with acute respiratory distress syndrome in humans. mBio 3:e00473-12. doi:10.1128/mBio.00473-12. - DOI - PMC - PubMed
Publication types
MeSH terms
Supplementary concepts
LinkOut - more resources
Full Text Sources
Medical
Miscellaneous