An Attenuated Zika Virus Encoding Non-Glycosylated Envelope (E) and Non-Structural Protein 1 (NS1) Confers Complete Protection against Lethal Challenge in a Mouse Model
- PMID: 31547297
- PMCID: PMC6789518
- DOI: 10.3390/vaccines7030112
An Attenuated Zika Virus Encoding Non-Glycosylated Envelope (E) and Non-Structural Protein 1 (NS1) Confers Complete Protection against Lethal Challenge in a Mouse Model
Abstract
Zika virus (ZIKV), a mosquito-transmitted flavivirus, emerged in the last decade causing serious human diseases, including congenital microcephaly in newborns and Guillain-Barré syndrome in adults. Although many vaccine platforms are at various stages of development, no licensed vaccines are currently available. Previously, we described a mutant MR766 ZIKV (m2MR) bearing an E protein mutation (N154A) that prevented its glycosylation, resulting in attenuation and defective neuroinvasion. To further attenuate m2MR for its potential use as a live viral vaccine, we incorporated additional mutations into m2MR by substituting the asparagine residues in the glycosylation sites (N130 and N207) of NS1 with alanine residues. Examination of pathogenic properties revealed that the virus (m5MR) carrying mutations in E (N154A) and NS1 (N130A and N207A) was fully attenuated with no disease signs in infected mice, inducing high levels of humoral and cell-mediated immune responses, and protecting mice from subsequent lethal virus challenge. Furthermore, passive transfer of sera from m5MR-infected mice into naïve animals resulted in complete protection from lethal challenge. The immune sera from m5MR-infected animals neutralized both African and Asian lineage viruses equally well, suggesting that m5MR virus could be developed as a potentially broad live virus vaccine candidate.
Keywords: NS1 protein; Zika virus; attenuation; glycosylation; vaccine candidate.
Conflict of interest statement
The authors declare no conflict of interest.
Figures
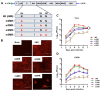
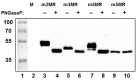
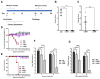
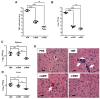
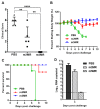
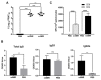
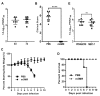
Similar articles
-
Zika Virus Encoding Nonglycosylated Envelope Protein Is Attenuated and Defective in Neuroinvasion.J Virol. 2017 Nov 14;91(23):e01348-17. doi: 10.1128/JVI.01348-17. Print 2017 Dec 1. J Virol. 2017. PMID: 28931684 Free PMC article.
-
N130, N175 and N207 are N-linked glycosylation sites of duck Tembusu virus NS1 that are important for viral multiplication, viremia and virulence in ducklings.Vet Microbiol. 2021 Oct;261:109215. doi: 10.1016/j.vetmic.2021.109215. Epub 2021 Aug 25. Vet Microbiol. 2021. PMID: 34455356
-
Zika Virus Attenuation by Codon Pair Deoptimization Induces Sterilizing Immunity in Mouse Models.J Virol. 2018 Aug 16;92(17):e00701-18. doi: 10.1128/JVI.00701-18. Print 2018 Sep 1. J Virol. 2018. PMID: 29925661 Free PMC article.
-
Zika virus structural biology and progress in vaccine development.Biotechnol Adv. 2018 Jan-Feb;36(1):47-53. doi: 10.1016/j.biotechadv.2017.09.004. Epub 2017 Sep 12. Biotechnol Adv. 2018. PMID: 28916391 Review.
-
The Dual Role of the Antibody Response Against the Flavivirus Non-structural Protein 1 (NS1) in Protection and Immuno-Pathogenesis.Front Immunol. 2019 Jul 18;10:1651. doi: 10.3389/fimmu.2019.01651. eCollection 2019. Front Immunol. 2019. PMID: 31379848 Free PMC article. Review.
Cited by
-
Redox Regulation and Metabolic Dependency of Zika Virus Replication: Inhibition by Nrf2-Antioxidant Response and NAD(H) Antimetabolites.J Virol. 2023 Feb 28;97(2):e0136322. doi: 10.1128/jvi.01363-22. Epub 2023 Jan 23. J Virol. 2023. PMID: 36688653 Free PMC article.
-
A Single Mutation at Position 120 in the Envelope Protein Attenuates Tembusu Virus in Ducks.Viruses. 2022 Feb 22;14(3):447. doi: 10.3390/v14030447. Viruses. 2022. PMID: 35336854 Free PMC article.
-
The Unique Glycosylation at Position 986 on the E2 Glycoprotein of Classical Swine Fever Virus Is Responsible for Viral Attenuation and Protection against Lethal Challenge.J Virol. 2022 Jan 26;96(2):e0176821. doi: 10.1128/JVI.01768-21. Epub 2021 Nov 3. J Virol. 2022. PMID: 34730400 Free PMC article.
-
Flavivirus NS1 and Its Potential in Vaccine Development.Vaccines (Basel). 2021 Jun 9;9(6):622. doi: 10.3390/vaccines9060622. Vaccines (Basel). 2021. PMID: 34207516 Free PMC article. Review.
-
Rational Development of Live-Attenuated Zika Virus Vaccines.Pathogens. 2023 Jan 28;12(2):194. doi: 10.3390/pathogens12020194. Pathogens. 2023. PMID: 36839466 Free PMC article. Review.
References
-
- Hills S.L., Russell K., Hennessey M., Williams C., Oster A.M., Fischer M., Mead P. Transmission of Zika Virus Through Sexual Contact with Travelers to Areas of Ongoing Transmission—Continental United States, 2016. MMWR Morb. Mortal. Wkly. Rep. 2016;65:215–216. doi: 10.15585/mmwr.mm6508e2. - DOI - PubMed
-
- Bailey M.J., Duehr J., Dulin H., Broecker F., Brown J.A., Arumemi F.O., Bermudez Gonzalez M.C., Leyva-Grado V.H., Evans M.J., Simon V., et al. Human antibodies targeting Zika virus NS1 provide protection against disease in a mouse model. Nat. Commun. 2018;9:4560. doi: 10.1038/s41467-018-07008-0. - DOI - PMC - PubMed
Grants and funding
LinkOut - more resources
Full Text Sources
Other Literature Sources
Research Materials