Modular enzyme assembly for enhanced cascade biocatalysis and metabolic flux
- PMID: 31534134
- PMCID: PMC6751169
- DOI: 10.1038/s41467-019-12247-w
Modular enzyme assembly for enhanced cascade biocatalysis and metabolic flux
Abstract
Enzymatic reactions in living cells are highly dynamic but simultaneously tightly regulated. Enzyme engineers seek to construct multienzyme complexes to prevent intermediate diffusion, to improve product yield, and to control the flux of metabolites. Here we choose a pair of short peptide tags (RIAD and RIDD) to create scaffold-free enzyme assemblies to achieve these goals. In vitro, assembling enzymes in the menaquinone biosynthetic pathway through RIAD-RIDD interaction yields protein nanoparticles with varying stoichiometries, sizes, geometries, and catalytic efficiency. In Escherichia coli, assembling the last enzyme of the upstream mevalonate pathway with the first enzyme of the downstream carotenoid pathway leads to the formation of a pathway node, which increases carotenoid production by 5.7 folds. The same strategy results in a 58% increase in lycopene production in engineered Saccharomyces cerevisiae. This work presents a simple strategy to impose metabolic control in biosynthetic microbe factories.
Conflict of interest statement
The authors declare no competing interests.
Figures
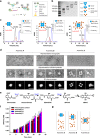
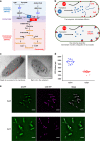
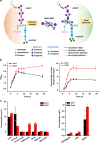
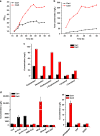
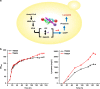
Similar articles
-
Multi-modular metabolic engineering and efflux engineering for enhanced lycopene production in recombinant Saccharomyces cerevisiae.J Ind Microbiol Biotechnol. 2024 Jan 9;51:kuae015. doi: 10.1093/jimb/kuae015. J Ind Microbiol Biotechnol. 2024. PMID: 38621758 Free PMC article.
-
CRISPR interference-guided balancing of a biosynthetic mevalonate pathway increases terpenoid production.Metab Eng. 2016 Nov;38:228-240. doi: 10.1016/j.ymben.2016.08.006. Epub 2016 Aug 26. Metab Eng. 2016. PMID: 27569599
-
Engineering a growth-phase-dependent biosynthetic pathway for carotenoid production in Saccharomyces cerevisiae.J Ind Microbiol Biotechnol. 2020 May;47(4-5):383-393. doi: 10.1007/s10295-020-02271-x. Epub 2020 Mar 31. J Ind Microbiol Biotechnol. 2020. PMID: 32236768
-
Metabolic Engineering Escherichia coli for the Production of Lycopene.Molecules. 2020 Jul 9;25(14):3136. doi: 10.3390/molecules25143136. Molecules. 2020. PMID: 32659911 Free PMC article. Review.
-
Microbial production of mevalonate.J Biotechnol. 2023 Jun 20;370:1-11. doi: 10.1016/j.jbiotec.2023.05.005. Epub 2023 May 18. J Biotechnol. 2023. PMID: 37209831 Review.
Cited by
-
Protein engineering for natural product biosynthesis and synthetic biology applications.Protein Eng Des Sel. 2021 Feb 15;34:gzab015. doi: 10.1093/protein/gzab015. Protein Eng Des Sel. 2021. PMID: 34137436 Free PMC article. Review.
-
De novo biosynthesis of τ-cadinol in engineered Escherichia coli.Bioresour Bioprocess. 2022 Mar 21;9(1):29. doi: 10.1186/s40643-022-00521-7. Bioresour Bioprocess. 2022. PMID: 38647768 Free PMC article.
-
Research progress of multi-enzyme complexes based on the design of scaffold protein.Bioresour Bioprocess. 2023 Oct 23;10(1):72. doi: 10.1186/s40643-023-00695-8. Bioresour Bioprocess. 2023. PMID: 38647916 Free PMC article. Review.
-
Construction of a Self-Purification and Self-Assembly Coenzyme Regeneration System for the Synthesis of Chiral Drug Intermediates.ACS Omega. 2021 Jan 13;6(3):1911-1916. doi: 10.1021/acsomega.0c04668. eCollection 2021 Jan 26. ACS Omega. 2021. PMID: 33521431 Free PMC article.
-
Modular engineering for microbial production of carotenoids.Metab Eng Commun. 2019 Dec 15;10:e00118. doi: 10.1016/j.mec.2019.e00118. eCollection 2020 Jun. Metab Eng Commun. 2019. PMID: 31908924 Free PMC article. Review.
References
Publication types
MeSH terms
Substances
LinkOut - more resources
Full Text Sources
Other Literature Sources
Molecular Biology Databases