Transposon-Mediated Horizontal Transfer of the Host-Specific Virulence Protein ToxA between Three Fungal Wheat Pathogens
- PMID: 31506307
- PMCID: PMC6737239
- DOI: 10.1128/mBio.01515-19
Transposon-Mediated Horizontal Transfer of the Host-Specific Virulence Protein ToxA between Three Fungal Wheat Pathogens
Abstract
Most known examples of horizontal gene transfer (HGT) between eukaryotes are ancient. These events are identified primarily using phylogenetic methods on coding regions alone. Only rarely are there examples of HGT where noncoding DNA is also reported. The gene encoding the wheat virulence protein ToxA and the surrounding 14 kb is one of these rare examples. ToxA has been horizontally transferred between three fungal wheat pathogens (Parastagonospora nodorum, Pyrenophora tritici-repentis, and Bipolaris sorokiniana) as part of a conserved ∼14 kb element which contains coding and noncoding regions. Here we used long-read sequencing to define the extent of HGT between these three fungal species. Construction of near-chromosomal-level assemblies enabled identification of terminal inverted repeats on either end of the 14 kb region, typical of a type II DNA transposon. This is the first description of ToxA with complete transposon features, which we call ToxhAT. In all three species, ToxhAT resides in a large (140-to-250 kb) transposon-rich genomic island which is absent in isolates that do not carry the gene (annotated here as toxa- ). We demonstrate that the horizontal transfer of ToxhAT between P. tritici-repentis and P. nodorum occurred as part of a large (∼80 kb) HGT which is now undergoing extensive decay. In B. sorokiniana, in contrast, ToxhAT and its resident genomic island are mobile within the genome. Together, these data provide insight into the noncoding regions that facilitate HGT between eukaryotes and into the genomic processes which mask the extent of HGT between these species.IMPORTANCE This work dissects the tripartite horizontal transfer of ToxA, a gene that has a direct negative impact on global wheat yields. Defining the extent of horizontally transferred DNA is important because it can provide clues to the mechanisms that facilitate HGT. Our analysis of ToxA and its surrounding 14 kb suggests that this gene was horizontally transferred in two independent events, with one event likely facilitated by a type II DNA transposon. These horizontal transfer events are now in various processes of decay in each species due to the repeated insertion of new transposons and subsequent rounds of targeted mutation by a fungal genome defense mechanism known as repeat induced point mutation. This work highlights the role that HGT plays in the evolution of host adaptation in eukaryotic pathogens. It also increases the growing body of evidence indicating that transposons facilitate adaptive HGT events between fungi present in similar environments and hosts.
Keywords: ToxA; adaptive evolution; fungal pathogen; fungal wheat pathogen; horizontal transfer; transposon; wheat pathogen.
Copyright © 2019 McDonald et al.
Figures
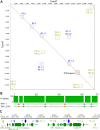
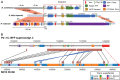
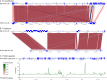
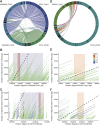
Similar articles
-
The pangenome of the wheat pathogen Pyrenophora tritici-repentis reveals novel transposons associated with necrotrophic effectors ToxA and ToxB.BMC Biol. 2022 Oct 24;20(1):239. doi: 10.1186/s12915-022-01433-w. BMC Biol. 2022. PMID: 36280878 Free PMC article.
-
Genomic distribution of a novel Pyrenophora tritici-repentis ToxA insertion element.PLoS One. 2018 Oct 31;13(10):e0206586. doi: 10.1371/journal.pone.0206586. eCollection 2018. PLoS One. 2018. PMID: 30379913 Free PMC article.
-
The discovery of the virulence gene ToxA in the wheat and barley pathogen Bipolaris sorokiniana.Mol Plant Pathol. 2018 Feb;19(2):432-439. doi: 10.1111/mpp.12535. Epub 2017 Mar 12. Mol Plant Pathol. 2018. PMID: 28093843 Free PMC article.
-
Horizontal gene and chromosome transfer in plant pathogenic fungi affecting host range.FEMS Microbiol Rev. 2011 May;35(3):542-54. doi: 10.1111/j.1574-6976.2010.00263.x. Epub 2011 Jan 26. FEMS Microbiol Rev. 2011. PMID: 21223323 Review.
-
Host-specific toxins: effectors of necrotrophic pathogenicity.Cell Microbiol. 2008 Jul;10(7):1421-8. doi: 10.1111/j.1462-5822.2008.01153.x. Epub 2008 Apr 1. Cell Microbiol. 2008. PMID: 18384660 Review.
Cited by
-
The pangenome of the wheat pathogen Pyrenophora tritici-repentis reveals novel transposons associated with necrotrophic effectors ToxA and ToxB.BMC Biol. 2022 Oct 24;20(1):239. doi: 10.1186/s12915-022-01433-w. BMC Biol. 2022. PMID: 36280878 Free PMC article.
-
Whole-genome sequence analysis of Bipolaris sorokiniana infecting wheat in India and characterization of ToxA gene in different isolates as pathogenicity determinants.3 Biotech. 2022 Jul;12(7):151. doi: 10.1007/s13205-022-03213-3. Epub 2022 Jun 20. 3 Biotech. 2022. PMID: 35747503 Free PMC article.
-
Dynamics in Secondary Metabolite Gene Clusters in Otherwise Highly Syntenic and Stable Genomes in the Fungal Genus Botrytis.Genome Biol Evol. 2020 Dec 6;12(12):2491-2507. doi: 10.1093/gbe/evaa218. Genome Biol Evol. 2020. PMID: 33283866 Free PMC article.
-
Increased Conidia Production and Germination In Vitro Correlate with Virulence Enhancement in Fusarium oxysporum f. sp. cucumerinum.J Fungi (Basel). 2023 Aug 14;9(8):847. doi: 10.3390/jof9080847. J Fungi (Basel). 2023. PMID: 37623618 Free PMC article.
-
Genome comparisons reveal accessory genes crucial for the evolution of apple Glomerella leaf spot pathogenicity in Colletotrichum fungi.Mol Plant Pathol. 2024 Apr;25(4):e13454. doi: 10.1111/mpp.13454. Mol Plant Pathol. 2024. PMID: 38619507 Free PMC article.
References
Publication types
MeSH terms
Substances
LinkOut - more resources
Full Text Sources
Other Literature Sources
Miscellaneous