Brain somatic mutations in MTOR reveal translational dysregulations underlying intractable focal epilepsy
- PMID: 31483294
- PMCID: PMC6763223
- DOI: 10.1172/JCI127032
Brain somatic mutations in MTOR reveal translational dysregulations underlying intractable focal epilepsy
Abstract
Brain somatic mutations confer genomic diversity in the human brain and cause neurodevelopmental disorders. Recently, brain somatic activating mutations in MTOR have been identified as a major etiology of intractable epilepsy in patients with cortical malformations. However, the molecular genetic mechanism of how brain somatic mutations in MTOR cause intractable epilepsy has remained elusive. In this study, translational profiling of intractable epilepsy mouse models with brain somatic mutations and genome-edited cells revealed a novel translational dysregulation mechanism and mTOR activation-sensitive targets mediated by human MTOR mutations that lead to intractable epilepsy with cortical malformation. These mTOR targets were found to be regulated by novel mTOR-responsive 5'-UTR motifs, distinct from known mTOR inhibition-sensitive targets regulated by 5' terminal oligopyrimidine motifs. Novel mTOR target genes were validated in patient brain tissues, and the mTOR downstream effector eIF4E was identified as a new therapeutic target in intractable epilepsy via pharmacological or genetic inhibition. We show that metformin, an FDA-approved eIF4E inhibitor, suppresses intractable epilepsy. Altogether, the present study describes translational dysregulation resulting from brain somatic mutations in MTOR, as well as the pathogenesis and potential therapeutic targets of intractable epilepsy.
Keywords: Epilepsy; Genetic variation; Neuroscience; Therapeutics; Translation.
Conflict of interest statement
Figures
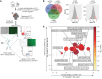
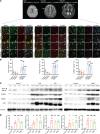
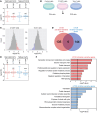
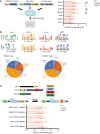
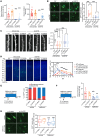
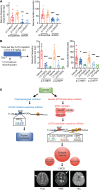
Similar articles
-
Somatic double-hit in MTOR and RPS6 in hemimegalencephaly with intractable epilepsy.Hum Mol Genet. 2019 Nov 15;28(22):3755-3765. doi: 10.1093/hmg/ddz194. Hum Mol Genet. 2019. PMID: 31411685 Free PMC article.
-
Brain somatic mutations in MTOR leading to focal cortical dysplasia.BMB Rep. 2016 Feb;49(2):71-2. doi: 10.5483/bmbrep.2016.49.2.010. BMB Rep. 2016. PMID: 26779999 Free PMC article.
-
Brain Somatic Mutations in MTOR Disrupt Neuronal Ciliogenesis, Leading to Focal Cortical Dyslamination.Neuron. 2018 Jul 11;99(1):83-97.e7. doi: 10.1016/j.neuron.2018.05.039. Epub 2018 Jun 21. Neuron. 2018. PMID: 29937275
-
mTOR pathway: Insights into an established pathway for brain mosaicism in epilepsy.Neurobiol Dis. 2023 Jun 15;182:106144. doi: 10.1016/j.nbd.2023.106144. Epub 2023 May 4. Neurobiol Dis. 2023. PMID: 37149062 Review.
-
Focal malformations of cortical development: new vistas for molecular pathogenesis.Neuroscience. 2013 Nov 12;252:262-76. doi: 10.1016/j.neuroscience.2013.07.037. Epub 2013 Jul 25. Neuroscience. 2013. PMID: 23892008 Review.
Cited by
-
InsP7 is a small-molecule regulator of NUDT3-mediated mRNA decapping and processing-body dynamics.Proc Natl Acad Sci U S A. 2020 Aug 11;117(32):19245-19253. doi: 10.1073/pnas.1922284117. Epub 2020 Jul 29. Proc Natl Acad Sci U S A. 2020. PMID: 32727897 Free PMC article.
-
Comprehensive Genome-Wide Approaches to Activity-Dependent Translational Control in Neurons.Int J Mol Sci. 2020 Feb 26;21(5):1592. doi: 10.3390/ijms21051592. Int J Mol Sci. 2020. PMID: 32111062 Free PMC article. Review.
-
Corrigendum: Convergent and Divergent Mechanisms of Epileptogenesis in mTORopathies.Front Neuroanat. 2021 Jul 6;15:715363. doi: 10.3389/fnana.2021.715363. eCollection 2021. Front Neuroanat. 2021. PMID: 34295225 Free PMC article.
-
The complex molecular epileptogenesis landscape of glioblastoma.Cell Rep Med. 2024 Aug 20;5(8):101691. doi: 10.1016/j.xcrm.2024.101691. Cell Rep Med. 2024. PMID: 39168100 Free PMC article.
-
Molecular regulation of brain metabolism underlying circadian epilepsy.Epilepsia. 2021 Feb;62 Suppl 1(Suppl 1):S32-S48. doi: 10.1111/epi.16796. Epub 2021 Jan 4. Epilepsia. 2021. PMID: 33395505 Free PMC article. Review.
References
Publication types
MeSH terms
Substances
LinkOut - more resources
Full Text Sources
Other Literature Sources
Molecular Biology Databases
Miscellaneous