Bisphosphonic Acid-Functionalized Cross-Linkers to Tailor Hydrogel Properties for Biomedical Applications
- PMID: 31458994
- PMCID: PMC6644954
- DOI: 10.1021/acsomega.8b01103
Bisphosphonic Acid-Functionalized Cross-Linkers to Tailor Hydrogel Properties for Biomedical Applications
Abstract
Two bisphosphonic acid-functionalized cross-linkers (one novel) with different spacer chain characteristics were synthesized and incorporated into hydrogels by copolymerization with 2-hydroxyethyl methacrylate at different ratios to control the hydrogels' swelling, mechanical properties, and ability to support mineralization for biomedical applications. The cross-linkers were synthesized by reaction of 2-isocyanatoethyl methacrylate and bisphosphonated diamines followed by selective dealkylation of the bisphosphonate ester groups. The hydrogels provide in vitro growth of carbonated apatite, morphology affected by the cross-linker structure. The hydrogels exhibit a high Young's modulus E (up to 400 kPa) and can sustain up to 10.2 ± 0.1 MPa compressive stresses. E and hence the cross-link density significantly increases upon mineralization reflecting the formation of many bisphosphonate BP-Ca2+ bonds acting as additional cross-links. Cyclic mechanical tests reveal self-recoverability of hydrogels because of reversible nature of BP-Ca2+ bonds. The results suggest that these cross-linkers can add calcium-binding abilities to hydrogels synthesized from any monomer and improve their mechanical, swelling, and mineralization properties and hence are potentially useful materials for biomedical applications.
Conflict of interest statement
The authors declare no competing financial interest.
Figures
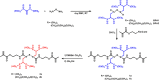
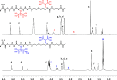
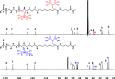
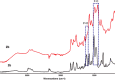
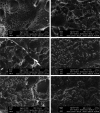
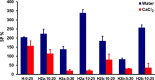
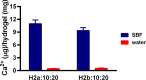
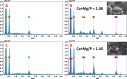
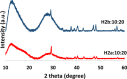
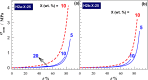
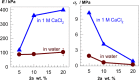
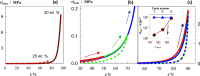
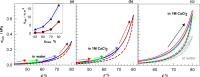
Similar articles
-
Stiff and Tough Hydrogels Prepared Through Integration of Ionic Cross-linking and Enzymatic Mineralization.Acta Biomater. 2022 Sep 1;149:220-232. doi: 10.1016/j.actbio.2022.06.008. Epub 2022 Jun 8. Acta Biomater. 2022. PMID: 35688402
-
Effect of sizes of vinyl modified narrow-dispersed silica cross-linker on the mechanical properties of acrylamide based hydrogel.Sci Rep. 2023 Mar 29;13(1):5089. doi: 10.1038/s41598-023-32185-4. Sci Rep. 2023. PMID: 36991034 Free PMC article.
-
Development of a Tough, Self-Healing Polyampholyte Terpolymer Hydrogel Patch with Enhanced Skin Adhesion via Tuning the Density and Strength of Ion-Pair Associations.ACS Appl Mater Interfaces. 2021 Feb 24;13(7):8889-8900. doi: 10.1021/acsami.0c19427. Epub 2021 Feb 15. ACS Appl Mater Interfaces. 2021. PMID: 33587615
-
Novel scaffolds based on poly(2-hydroxyethyl methacrylate) superporous hydrogels for bone tissue engineering.J Biomater Sci Polym Ed. 2011;22(9):1157-78. doi: 10.1163/092050610X501704. J Biomater Sci Polym Ed. 2011. PMID: 20615330
-
Double-Network Tough Hydrogels: A Brief Review on Achievements and Challenges.Gels. 2022 Apr 18;8(4):247. doi: 10.3390/gels8040247. Gels. 2022. PMID: 35448148 Free PMC article. Review.
Cited by
-
Controlled-release of apatinib for targeted inhibition of osteosarcoma by supramolecular nanovalve-modified mesoporous silica.Front Bioeng Biotechnol. 2023 Feb 16;11:1135655. doi: 10.3389/fbioe.2023.1135655. eCollection 2023. Front Bioeng Biotechnol. 2023. PMID: 36873361 Free PMC article.
-
Antimicrobial Peptide-Polymer Conjugates for Dentistry.ACS Appl Polym Mater. 2020 Mar 13;2(3):1134-1144. doi: 10.1021/acsapm.9b00921. Epub 2020 Jan 2. ACS Appl Polym Mater. 2020. PMID: 33834166 Free PMC article.
-
Novel Black Seed Polysaccharide Extract-g-Poly (Acrylate) pH-Responsive Hydrogel Nanocomposites for Safe Oral Insulin Delivery: Development, In Vitro, In Vivo and Toxicological Evaluation.Pharmaceutics. 2022 Dec 25;15(1):62. doi: 10.3390/pharmaceutics15010062. Pharmaceutics. 2022. PMID: 36678691 Free PMC article.
References
-
- Caló E.; Khutoryanskiy V. V. Biomedical applications of hydrogels: A review of patents and commercial products. Eur. Polym. J. 2015, 65, 252–267. 10.1016/j.eurpolymj.2014.11.024. - DOI
-
- Chirila T. V.; Zainuddin Calcification of synthetic polymers functionalized with negatively ionizable groups: A critical review. React. Funct. Polym. 2007, 67, 165–172. 10.1016/j.reactfunctpolym.2006.10.008. - DOI
LinkOut - more resources
Full Text Sources