Herpes Simplex Virus, Alzheimer's Disease and a Possible Role for Rab GTPases
- PMID: 31448273
- PMCID: PMC6692634
- DOI: 10.3389/fcell.2019.00134
Herpes Simplex Virus, Alzheimer's Disease and a Possible Role for Rab GTPases
Abstract
Herpes simplex virus (HSV) is a common pathogen, infecting 85% of adults in the United States. After reaching the nucleus of the long-lived neuron, HSV may enter latency to persist throughout the life span. Re-activation of latent herpesviruses is associated with progressive cognitive impairment and Alzheimer's disease (AD). As an enveloped DNA virus, HSV exploits cellular membrane systems for its life cycle, and thereby comes in contact with the Rab family of GTPases, master regulators of intracellular membrane dynamics. Knock-down and overexpression of specific Rabs reduce HSV production. Disheveled membrane compartments could lead to AD because membrane sorting and trafficking are crucial for synaptic vesicle formation, neuronal survival signaling and Abeta production. Amyloid precursor protein (APP), a transmembrane glycoprotein, is the parent of Abeta, the major component of senile plaques in AD. Up-regulation of APP expression due to HSV is significant since excess APP interferes with Rab5 endocytic trafficking in neurons. Here, we show that purified PC12-cell endosomes transport both anterograde and retrograde when injected into the squid giant axon at rates similar to isolated HSV. Intracellular HSV co-fractionates with these endosomes, contains APP, Rab5 and TrkA, and displays a second membrane. HSV infected PC12 cells up-regulate APP expression. Whether interference with Rabs has a specific effect on HSV or indirectly affects membrane compartment dynamics co-opted by virus needs further study. Ultimately Rabs, their effectors or their membrane-binding partners may serve as handles to reduce the impact of viral re-activation on cognitive function, or even as more general-purpose anti-microbial therapies.
Keywords: Alzheimer’s disease; HSV (herpes simplex virus); Rab GTPases; axonal transport; dynein; endosomes; kinesin; squid giant axon.
Figures
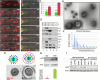
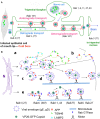
Similar articles
-
Herpes Simplex Virus gE/gI and US9 Promote both Envelopment and Sorting of Virus Particles in the Cytoplasm of Neurons, Two Processes That Precede Anterograde Transport in Axons.J Virol. 2017 May 12;91(11):e00050-17. doi: 10.1128/JVI.00050-17. Print 2017 Jun 1. J Virol. 2017. PMID: 28331094 Free PMC article.
-
Role of Rab GTPases in HSV-1 infection: Molecular understanding of viral maturation and egress.Microb Pathog. 2018 May;118:146-153. doi: 10.1016/j.micpath.2018.03.028. Epub 2018 Mar 16. Microb Pathog. 2018. PMID: 29551438 Review.
-
Fast anterograde transport of herpes simplex virus: role for the amyloid precursor protein of alzheimer's disease.Aging Cell. 2003 Dec;2(6):305-18. doi: 10.1046/j.1474-9728.2003.00069.x. Aging Cell. 2003. PMID: 14677633 Free PMC article.
-
Kinesin-1 Proteins KIF5A, -5B, and -5C Promote Anterograde Transport of Herpes Simplex Virus Enveloped Virions in Axons.J Virol. 2018 Sep 26;92(20):e01269-18. doi: 10.1128/JVI.01269-18. Print 2018 Oct 15. J Virol. 2018. PMID: 30068641 Free PMC article.
-
Molecular Mechanisms for Herpes Simplex Virus Type 1 Pathogenesis in Alzheimer's Disease.Front Aging Neurosci. 2018 Mar 6;10:48. doi: 10.3389/fnagi.2018.00048. eCollection 2018. Front Aging Neurosci. 2018. PMID: 29559905 Free PMC article. Review.
Cited by
-
Therapeutic Targeting of Rab GTPases: Relevance for Alzheimer's Disease.Biomedicines. 2022 May 16;10(5):1141. doi: 10.3390/biomedicines10051141. Biomedicines. 2022. PMID: 35625878 Free PMC article. Review.
-
Decoupling the Effects of the Amyloid Precursor Protein From Amyloid-β Plaques on Axonal Transport Dynamics in the Living Brain.Front Cell Neurosci. 2019 Dec 3;13:501. doi: 10.3389/fncel.2019.00501. eCollection 2019. Front Cell Neurosci. 2019. PMID: 31849608 Free PMC article.
-
Molecular Level Insight Into the Benefit of Myricetin and Dihydromyricetin Uptake in Patients With Alzheimer's Diseases.Front Aging Neurosci. 2020 Oct 23;12:601603. doi: 10.3389/fnagi.2020.601603. eCollection 2020. Front Aging Neurosci. 2020. PMID: 33192493 Free PMC article. Review.
-
Harnessing axonal transport to map reward circuitry: Differing brain-wide projections from medial prefrontal cortical domains.Front Cell Dev Biol. 2023 Nov 30;11:1278831. doi: 10.3389/fcell.2023.1278831. eCollection 2023. Front Cell Dev Biol. 2023. PMID: 38099294 Free PMC article.
-
Modulation of Endosome Function, Vesicle Trafficking and Autophagy by Human Herpesviruses.Cells. 2021 Mar 4;10(3):542. doi: 10.3390/cells10030542. Cells. 2021. PMID: 33806291 Free PMC article. Review.
References
-
- Agola J. O., Sivalingam D., Cimino D. F., Simons P. C., Buranda T., Sklar L. A., et al. (2015). Quantitative bead-based flow cytometry for assaying Rab7 GTPase interaction with the Rab-interacting lysosomal protein (RILP) effector protein. Methods Mol. Biol. 1298 331–354. 10.1007/978-1-4939-2569-8_28 - DOI - PMC - PubMed
Grants and funding
LinkOut - more resources
Full Text Sources
Research Materials