Extracellular Nucleotides and P2 Receptors in Renal Function
- PMID: 31437091
- PMCID: PMC6985785
- DOI: 10.1152/physrev.00038.2018
Extracellular Nucleotides and P2 Receptors in Renal Function
Abstract
The understanding of the nucleotide/P2 receptor system in the regulation of renal hemodynamics and transport function has grown exponentially over the last 20 yr. This review attempts to integrate the available data while also identifying areas of missing information. First, the determinants of nucleotide concentrations in the interstitial and tubular fluids of the kidney are described, including mechanisms of cellular release of nucleotides and their extracellular breakdown. Then the renal cell membrane expression of P2X and P2Y receptors is discussed in the context of their effects on renal vascular and tubular functions. Attention is paid to effects on the cortical vasculature and intraglomerular structures, autoregulation of renal blood flow, tubuloglomerular feedback, and the control of medullary blood flow. The role of the nucleotide/P2 receptor system in the autocrine/paracrine regulation of sodium and fluid transport in the tubular and collecting duct system is outlined together with its role in integrative sodium and fluid homeostasis and blood pressure control. The final section summarizes the rapidly growing evidence indicating a prominent role of the extracellular nucleotide/P2 receptor system in the pathophysiology of the kidney and aims to identify potential therapeutic opportunities, including hypertension, lithium-induced nephropathy, polycystic kidney disease, and kidney inflammation. We are only beginning to unravel the distinct physiological and pathophysiological influences of the extracellular nucleotide/P2 receptor system and the associated therapeutic perspectives.
Keywords: P2 receptors; kidney; nucleotides; renal hemodynamics; transport function.
Conflict of interest statement
Over the past 36 mo, V. Vallon has served as a consultant and received honoraria from Astra-Zeneca, Bayer, Boehringer Ingelheim, Janssen Pharmaceutical, Eli Lilly, and Merck and has received grant support for investigator-initiated research from Astra-Zeneca, Bayer, Boehringer Ingelheim, Fresenius, and Janssen. R. Unwin is an employee of AstraZeneca IMED ECD CVRM (Gothenburg, Sweden). B. K. Kishore received research grant support and is collaborating with AstraZeneca and has United States patents issued for the use of P2Y2 and P2Y12 antagonists for the treatment of nephrogenic diabetes insipidus. E. W. Inscho and J. Leipziger have no conflicts of interest, financial or otherwise.
Figures
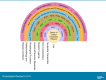
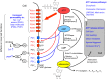
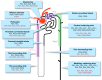
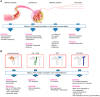
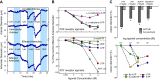
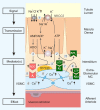
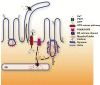
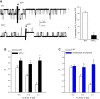
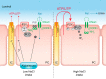
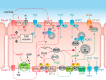
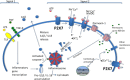
Similar articles
-
P2 receptors in the regulation of renal transport mechanisms.Am J Physiol Renal Physiol. 2008 Jan;294(1):F10-27. doi: 10.1152/ajprenal.00432.2007. Epub 2007 Oct 31. Am J Physiol Renal Physiol. 2008. PMID: 17977905 Review.
-
Purinergic signaling in kidney disease.Kidney Int. 2017 Feb;91(2):315-323. doi: 10.1016/j.kint.2016.08.029. Epub 2016 Oct 22. Kidney Int. 2017. PMID: 27780585 Review.
-
Purinergic signalling in the kidney: In physiology and disease.Biochem Pharmacol. 2021 May;187:114389. doi: 10.1016/j.bcp.2020.114389. Epub 2020 Dec 31. Biochem Pharmacol. 2021. PMID: 33359067 Review.
-
Role of adenosine 5'-triphosphate in regulating renal microvascular function and in hypertension.Hypertension. 2011 Sep;58(3):333-40. doi: 10.1161/HYPERTENSIONAHA.110.155952. Epub 2011 Jul 18. Hypertension. 2011. PMID: 21768526 Free PMC article. Review.
-
Effects of luminal flow and nucleotides on [Ca(2+)](i) in rabbit cortical collecting duct.Am J Physiol Renal Physiol. 2002 Sep;283(3):F437-46. doi: 10.1152/ajprenal.00316.2001. Am J Physiol Renal Physiol. 2002. PMID: 12167594
Cited by
-
Purinergic signaling in the male reproductive tract.Front Endocrinol (Lausanne). 2022 Nov 7;13:1049511. doi: 10.3389/fendo.2022.1049511. eCollection 2022. Front Endocrinol (Lausanne). 2022. PMID: 36419764 Free PMC article. Review.
-
P2 X 7 receptor is a critical regulator of extracellular ATP-induced profibrotic genes expression in rat kidney: implication of transforming growth factor-β/Smad signaling pathway.Purinergic Signal. 2024 Aug;20(4):421-430. doi: 10.1007/s11302-023-09977-4. Epub 2023 Nov 7. Purinergic Signal. 2024. PMID: 37934321 Free PMC article.
-
How Does Aldosterone Work in the β-Intercalated Cell?J Am Soc Nephrol. 2020 Mar;31(3):451-452. doi: 10.1681/ASN.2020010077. Epub 2020 Feb 13. J Am Soc Nephrol. 2020. PMID: 32065116 Free PMC article. No abstract available.
-
Purinoceptors, renal microvascular function and hypertension.Physiol Res. 2020 Jul 16;69(3):353-369. doi: 10.33549/physiolres.934463. Epub 2020 Apr 17. Physiol Res. 2020. PMID: 32301620 Free PMC article. Review.
-
Calcium signalling and transport in the kidney.Nat Rev Nephrol. 2024 Aug;20(8):541-555. doi: 10.1038/s41581-024-00835-z. Epub 2024 Apr 19. Nat Rev Nephrol. 2024. PMID: 38641658 Review.
References
-
- Abbracchio MP, Boeynaems JM, Barnard EA, Boyer JL, Kennedy C, Miras-Portugal MT, King BF, Gachet C, Jacobson KA, Weisman GA, Burnstock G. Characterization of the UDP-glucose receptor (re-named here the P2Y14 receptor) adds diversity to the P2Y receptor family. Trends Pharmacol Sci 24: 52–55, 2003. doi:10.1016/S0165-6147(02)00038-X. - DOI - PMC - PubMed
-
- Abbracchio MP, Burnstock G, Boeynaems JM, Barnard EA, Boyer JL, Kennedy C, Knight GE, Fumagalli M, Gachet C, Jacobson KA, Weisman GA. International Union of Pharmacology LVIII: update on the P2Y G protein-coupled nucleotide receptors: from molecular mechanisms and pathophysiology to therapy. Pharmacol Rev 58: 281–341, 2006. doi:10.1124/pr.58.3.3. - DOI - PMC - PubMed
-
- Abdelrahman A, Namasivayam V, Hinz S, Schiedel AC, Köse M, Burton M, El-Tayeb A, Gillard M, Bajorath J, de Ryck M, Müller CE. Characterization of P2X4 receptor agonists and antagonists by calcium influx and radioligand binding studies. Biochem Pharmacol 125: 41–54, 2017. doi:10.1016/j.bcp.2016.11.016. - DOI - PubMed
Publication types
MeSH terms
Substances
Grants and funding
LinkOut - more resources
Full Text Sources