Autophagy modulation in bladder cancer development and treatment (Review)
- PMID: 31436298
- PMCID: PMC6775810
- DOI: 10.3892/or.2019.7286
Autophagy modulation in bladder cancer development and treatment (Review)
Abstract
Bladder cancer (BC) is a potentially life‑threatening malignancy. Due to a high recurrence rate, frequent surveillance strategies and intravesical drug therapies, BC is considered one of the most expensive tumors to treat. As a fundamental evolutionary catabolic process, autophagy plays an important role in the maintenance of cellular environmental homeostasis by degrading and recycling damaged cytoplasmic components, including macromolecules and organelles. Scientific studies in the last two decades have shown that autophagy acts as a double‑edged sword with regard to the treatment of cancer. On one hand, autophagy inhibition is able to increase the sensitivity of cancer cells to treatment, a process known as protective autophagy. On the other hand, autophagy overactivation may lead to cell death, referred to as autophagic cell death, similar to apoptosis. Therefore, it is essential to identify the role of autophagy in cancer cells in order to develop novel therapeutic agents. In addition, autophagy may potentially become a novel therapeutic target in human diseases. In this review, the current knowledge on autophagy modulation in BC development and treatment is summarized.
Figures
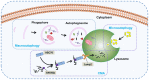
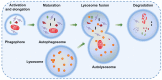
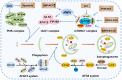
Similar articles
-
Therapeutic strategies of drug repositioning targeting autophagy to induce cancer cell death: from pathophysiology to treatment.J Hematol Oncol. 2017 Mar 9;10(1):67. doi: 10.1186/s13045-017-0436-9. J Hematol Oncol. 2017. PMID: 28279189 Free PMC article. Review.
-
Autophagy induction enhances tetrandrine-induced apoptosis via the AMPK/mTOR pathway in human bladder cancer cells.Oncol Rep. 2017 Nov;38(5):3137-3143. doi: 10.3892/or.2017.5988. Epub 2017 Sep 21. Oncol Rep. 2017. PMID: 29048631
-
Contrast effects of autophagy in the treatment of bladder cancer.Exp Biol Med (Maywood). 2021 Feb;246(3):354-367. doi: 10.1177/1535370220959336. Epub 2020 Sep 20. Exp Biol Med (Maywood). 2021. PMID: 32954815 Free PMC article. Review.
-
Pirarubicin induces an autophagic cytoprotective response through suppression of the mammalian target of rapamycin signaling pathway in human bladder cancer cells.Biochem Biophys Res Commun. 2015 May 1;460(2):380-5. doi: 10.1016/j.bbrc.2015.03.042. Epub 2015 Mar 17. Biochem Biophys Res Commun. 2015. PMID: 25791481
-
Targeting the prodeath and prosurvival functions of autophagy as novel therapeutic strategies in cancer.Autophagy. 2010 Apr;6(3):322-9. doi: 10.4161/auto.6.3.11625. Epub 2010 Apr 26. Autophagy. 2010. PMID: 20224296 Free PMC article. Review.
Cited by
-
Activation of autophagy triggers mitochondrial loss and changes acetylation profile relevant for mechanotransduction in bladder cancer cells.Arch Toxicol. 2023 Jan;97(1):217-233. doi: 10.1007/s00204-022-03375-2. Epub 2022 Oct 10. Arch Toxicol. 2023. PMID: 36214828 Free PMC article.
-
An autophagy-related long non-coding RNA prognostic signature accurately predicts survival outcomes in bladder urothelial carcinoma patients.Aging (Albany NY). 2020 Aug 29;12(15):15624-15637. doi: 10.18632/aging.103718. Aging (Albany NY). 2020. PMID: 32805727 Free PMC article.
-
Bladder Cancer-related microRNAs With In Vivo Efficacy in Preclinical Models.Cancer Diagn Progn. 2021 Jul 3;1(4):245-263. doi: 10.21873/cdp.10033. eCollection 2021 Sep-Oct. Cancer Diagn Progn. 2021. PMID: 35403137 Free PMC article. Review.
-
Ehrlichia effector SLiM-icry: Artifice of cellular subversion.Front Cell Infect Microbiol. 2023 Mar 7;13:1150758. doi: 10.3389/fcimb.2023.1150758. eCollection 2023. Front Cell Infect Microbiol. 2023. PMID: 36960039 Free PMC article. Review.
-
The new horizon of biomarker in melanoma patients: A study based on autophagy-related long non-coding RNA.Medicine (Baltimore). 2022 Jan 7;101(1):e28553. doi: 10.1097/MD.0000000000028553. Medicine (Baltimore). 2022. PMID: 35029926 Free PMC article.
References
-
- Steurer S, Singer JM, Rink M, Chun F, Dahlem R, Simon R, Burandt E, Stahl P, Terracciano L, Schlomm T, et al. MALDI imaging-based identification of prognostically relevant signals in bladder cancer using large-scale tissue microarrays. Urol Oncol. 2014;32:1225–1233. doi: 10.1016/j.urolonc.2014.06.007. - DOI - PubMed
Publication types
MeSH terms
LinkOut - more resources
Full Text Sources
Medical
Research Materials