Exploration of Plant-Microbe Interactions for Sustainable Agriculture in CRISPR Era
- PMID: 31426522
- PMCID: PMC6723455
- DOI: 10.3390/microorganisms7080269
Exploration of Plant-Microbe Interactions for Sustainable Agriculture in CRISPR Era
Abstract
Plants and microbes are co-evolved and interact with each other in nature. Plant-associated microbes, often referred to as plant microbiota, are an integral part of plant life. Depending on the health effects on hosts, plant-microbe (PM) interactions are either beneficial or harmful. The role of microbiota in plant growth promotion (PGP) and protection against various stresses is well known. Recently, our knowledge of community composition of plant microbiome and significant driving factors have significantly improved. So, the use of plant microbiome is a reliable approach for a next green revolution and to meet the global food demand in sustainable and eco-friendly agriculture. An application of the multifaceted PM interactions needs the use of novel tools to know critical genetic and molecular aspects. Recently discovered clustered regularly interspaced short palindromic repeats (CRISPR)/Cas-mediated genome editing (GE) tools are of great interest to explore PM interactions. A systematic understanding of the PM interactions will enable the application of GE tools to enhance the capacity of microbes or plants for agronomic trait improvement. This review focuses on applying GE techniques in plants or associated microbiota for discovering the fundamentals of the PM interactions, disease resistance, PGP activity, and future implications in agriculture.
Keywords: CRISPR/Cas; genome editing; plant disease resistance; plant growth promotion; plant microbiome.
Conflict of interest statement
The authors declare no conflict of interest that might be perceived as affecting the objectivity of this review.
Figures
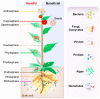
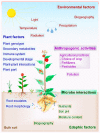
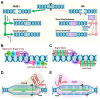
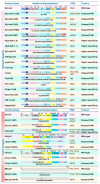
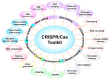
Similar articles
-
Application of CRISPR-Cas9 in plant-plant growth-promoting rhizobacteria interactions for next Green Revolution.3 Biotech. 2021 Dec;11(12):492. doi: 10.1007/s13205-021-03041-x. Epub 2021 Nov 13. 3 Biotech. 2021. PMID: 34840925 Free PMC article. Review.
-
Understanding the Plant-microbe Interactions in CRISPR/CAS9 Era: Indeed a Sprinting Start in Marathon.Curr Genomics. 2020 Sep;21(6):429-443. doi: 10.2174/1389202921999200716110853. Curr Genomics. 2020. PMID: 33093805 Free PMC article. Review.
-
Crop microbiome: their role and advances in molecular and omic techniques for the sustenance of agriculture.Planta. 2022 Dec 30;257(2):27. doi: 10.1007/s00425-022-04052-5. Planta. 2022. PMID: 36583789 Review.
-
Current technological interventions and applications of CRISPR/Cas for crop improvement.Mol Biol Rep. 2022 Jun;49(6):5751-5770. doi: 10.1007/s11033-021-06926-5. Epub 2021 Nov 22. Mol Biol Rep. 2022. PMID: 34807378 Review.
-
Plant Viruses: From Targets to Tools for CRISPR.Viruses. 2021 Jan 19;13(1):141. doi: 10.3390/v13010141. Viruses. 2021. PMID: 33478128 Free PMC article. Review.
Cited by
-
Biofertilizers and Biocontrol Agents for Agriculture: How to Identify and Develop New Potent Microbial Strains and Traits.Microorganisms. 2021 Apr 13;9(4):817. doi: 10.3390/microorganisms9040817. Microorganisms. 2021. PMID: 33924411 Free PMC article. Review.
-
Delineation of mechanistic approaches of rhizosphere microorganisms facilitated plant health and resilience under challenging conditions.3 Biotech. 2022 Mar;12(3):57. doi: 10.1007/s13205-022-03115-4. Epub 2022 Feb 4. 3 Biotech. 2022. PMID: 35186654 Free PMC article. Review.
-
Prime Editing Technology and Its Prospects for Future Applications in Plant Biology Research.Biodes Res. 2020 Jun 26;2020:9350905. doi: 10.34133/2020/9350905. eCollection 2020. Biodes Res. 2020. PMID: 37849904 Free PMC article. Review.
-
Distinct rhizosphere soil responses to nitrogen in relation to microbial biomass and community composition at initial flowering stages of alfalfa cultivars.Front Plant Sci. 2022 Aug 24;13:938865. doi: 10.3389/fpls.2022.938865. eCollection 2022. Front Plant Sci. 2022. PMID: 36092415 Free PMC article.
-
Optical Sensing Technologies to Elucidate the Interplay between Plant and Microbes.Micromachines (Basel). 2023 Jan 12;14(1):195. doi: 10.3390/mi14010195. Micromachines (Basel). 2023. PMID: 36677256 Free PMC article. Review.
References
-
- Kirjavainen P.V., Karvonen A.M., Adams R.I., Täubel M., Roponen M., Tuoresmäki P., Loss G., Jayaprakash B., Depner M., Ege M.J., et al. Farm-like indoor microbiota in non-farm homes protects children from asthma development. Nat. Med. 2019;25:1089–1095. doi: 10.1038/s41591-019-0469-4. - DOI - PubMed
-
- Waghunde R.R., Shelake R.M., Shinde M.S., Hayashi H. Microorganisms for Green Revolution. Springer; Singapore: 2017. Endophyte Microbes: A Weapon for Plant Health Management; pp. 303–325.
Publication types
Grants and funding
LinkOut - more resources
Full Text Sources
Miscellaneous