Compartmentalized Synthesis of Triacylglycerol at the Inner Nuclear Membrane Regulates Nuclear Organization
- PMID: 31422915
- PMCID: PMC6859503
- DOI: 10.1016/j.devcel.2019.07.009
Compartmentalized Synthesis of Triacylglycerol at the Inner Nuclear Membrane Regulates Nuclear Organization
Abstract
Cells dynamically adjust organelle organization in response to growth and environmental cues. This requires regulation of synthesis of phospholipids, the building blocks of organelle membranes, or remodeling of their fatty-acyl (FA) composition. FAs are also the main components of triacyglycerols (TGs), which enable energy storage in lipid droplets. How cells coordinate FA metabolism with organelle biogenesis during cell growth remains unclear. Here, we show that Lro1, an acyltransferase that generates TGs from phospholipid-derived FAs in yeast, relocates from the endoplasmic reticulum to a subdomain of the inner nuclear membrane. Lro1 nuclear targeting is regulated by cell cycle and nutrient starvation signals and is inhibited when the nucleus expands. Lro1 is active at this nuclear subdomain, and its compartmentalization is critical for nuclear integrity. These data suggest that Lro1 nuclear targeting provides a site of TG synthesis, which is coupled with nuclear membrane remodeling.
Keywords: lipid droplet; nuclear membrane; nucleus; phospholipid; triglyceride.
Copyright © 2019 The Authors. Published by Elsevier Inc. All rights reserved.
Conflict of interest statement
The authors declare no competing interests.
Figures
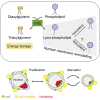
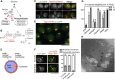
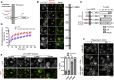
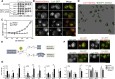
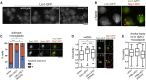
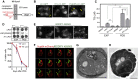
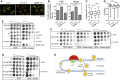
Similar articles
-
Depressing time: Waiting, melancholia, and the psychoanalytic practice of care.In: Kirtsoglou E, Simpson B, editors. The Time of Anthropology: Studies of Contemporary Chronopolitics. Abingdon: Routledge; 2020. Chapter 5. In: Kirtsoglou E, Simpson B, editors. The Time of Anthropology: Studies of Contemporary Chronopolitics. Abingdon: Routledge; 2020. Chapter 5. PMID: 36137063 Free Books & Documents. Review.
-
Qualitative evidence synthesis informing our understanding of people's perceptions and experiences of targeted digital communication.Cochrane Database Syst Rev. 2019 Oct 23;10(10):ED000141. doi: 10.1002/14651858.ED000141. Cochrane Database Syst Rev. 2019. PMID: 31643081 Free PMC article.
-
Comparison of Two Modern Survival Prediction Tools, SORG-MLA and METSSS, in Patients With Symptomatic Long-bone Metastases Who Underwent Local Treatment With Surgery Followed by Radiotherapy and With Radiotherapy Alone.Clin Orthop Relat Res. 2024 Dec 1;482(12):2193-2208. doi: 10.1097/CORR.0000000000003185. Epub 2024 Jul 23. Clin Orthop Relat Res. 2024. PMID: 39051924
-
Using Experience Sampling Methodology to Capture Disclosure Opportunities for Autistic Adults.Autism Adulthood. 2023 Dec 1;5(4):389-400. doi: 10.1089/aut.2022.0090. Epub 2023 Dec 12. Autism Adulthood. 2023. PMID: 38116059 Free PMC article.
-
Trends in Surgical and Nonsurgical Aesthetic Procedures: A 14-Year Analysis of the International Society of Aesthetic Plastic Surgery-ISAPS.Aesthetic Plast Surg. 2024 Oct;48(20):4217-4227. doi: 10.1007/s00266-024-04260-2. Epub 2024 Aug 5. Aesthetic Plast Surg. 2024. PMID: 39103642 Review.
Cited by
-
Regulation of organelle size and organization during development.Semin Cell Dev Biol. 2023 Jan 15;133:53-64. doi: 10.1016/j.semcdb.2022.02.002. Epub 2022 Feb 8. Semin Cell Dev Biol. 2023. PMID: 35148938 Free PMC article. Review.
-
SIR telomere silencing depends on nuclear envelope lipids and modulates sensitivity to a lysolipid.J Cell Biol. 2023 Jul 3;222(7):e202206061. doi: 10.1083/jcb.202206061. Epub 2023 Apr 12. J Cell Biol. 2023. PMID: 37042812 Free PMC article.
-
SUNny Ways: The Role of the SUN-Domain Protein Mps3 Bridging Yeast Nuclear Organization and Lipid Homeostasis.Front Genet. 2020 Feb 28;11:136. doi: 10.3389/fgene.2020.00136. eCollection 2020. Front Genet. 2020. PMID: 32184804 Free PMC article. Review.
-
The partitioning of fatty acids between membrane and storage lipids controls ER membrane expansion.bioRxiv [Preprint]. 2024 Sep 5:2024.09.05.611378. doi: 10.1101/2024.09.05.611378. bioRxiv. 2024. Update in: EMBO J. 2025 Jan 3. doi: 10.1038/s44318-024-00355-3 PMID: 39282465 Free PMC article. Updated. Preprint.
-
Reprogrammed lipid metabolism protects inner nuclear membrane against unsaturated fat.Dev Cell. 2021 Sep 27;56(18):2562-2578.e3. doi: 10.1016/j.devcel.2021.07.018. Epub 2021 Aug 17. Dev Cell. 2021. PMID: 34407429 Free PMC article.
References
-
- Albert B., Knight B., Merwin J., Martin V., Ottoz D., Gloor Y., Bruzzone M.J., Rudner A., Shore D. A molecular titration system coordinates ribosomal protein gene transcription with ribosomal RNA synthesis. Mol. Cell. 2016;64:720–733. - PubMed
-
- Barbosa A.D., Siniossoglou S. Function of lipid droplet-organelle interactions in lipid homeostasis. Biochim. Biophys. Acta. 2017;1864:1459–1468. - PubMed
Publication types
MeSH terms
Substances
Grants and funding
LinkOut - more resources
Full Text Sources
Other Literature Sources
Molecular Biology Databases
Research Materials
Miscellaneous