A tetraspanin gene regulating auxin response and affecting orchid perianth size and various plant developmental processes
- PMID: 31406958
- PMCID: PMC6680136
- DOI: 10.1002/pld3.157
A tetraspanin gene regulating auxin response and affecting orchid perianth size and various plant developmental processes
Abstract
The competition between L (lip) and SP (sepal/petal) complexes in P-code model determines the identity of complex perianth patterns in orchids. Orchid tetraspanin gene Auxin Activation Factor (AAF) orthologs, whose expression strongly correlated with the expansion and size of the perianth after P code established, were identified. Virus-induced gene silencing (VIGS) of OAGL6-2 in L complex resulted in smaller lips and the down-regulation of Oncidium OnAAF. VIGS of PeMADS9 in L complex resulted in the enlarged lips and up-regulation of Phalaenopsis PaAAF. Furthermore, the larger size of Phalaenopsis variety flowers was associated with higher PaAAF expression, larger and more cells in the perianth. Thus, a rule is established that whenever bigger perianth organs are made in orchids, higher OnAAF/PaAAF expression is observed after their identities are determined by P-code complexes. Ectopic expression Arabidopsis AtAAF significantly increased the size of flower organs by promoting cell expansion in transgenic Arabidopsis due to the enhancement of the efficiency of the auxin response and the subsequent suppression of the jasmonic acid (JA) biosynthesis genes (DAD1/OPR3) and BIGPETAL gene during late flower development. In addition, auxin-controlled phenotypes, such as indehiscent anthers, enhanced drought tolerance, and increased lateral root formation, were also observed in 35S::AtAAF plants. Furthermore, 35S::AtAAF root tips maintained gravitropism during auxin treatment. In contrast, the opposite phenotype was observed in palmitoylation-deficient AtAAF mutants. Our data demonstrate an interaction between the tetraspanin AAF and auxin/JA that regulates the size of flower organs and impacts various developmental processes.
Keywords: Arabidopsis thaliana; auxin response; orchids; perianth; tetraspanin.
Conflict of interest statement
The authors declare no conflict of interest associated with the work described in this manuscript.
Figures
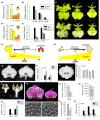
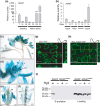
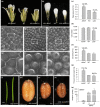
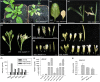
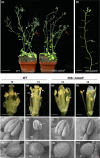
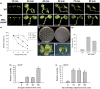
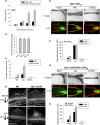
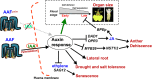

Similar articles
-
PaWOX3 and PaWOX3B Regulate Flower Number and the Lip Symmetry of Phalaenopsis.Plant Cell Physiol. 2024 Sep 3;65(8):1328-1343. doi: 10.1093/pcp/pcae069. Plant Cell Physiol. 2024. PMID: 38903045
-
Auxin controls Arabidopsis anther dehiscence by regulating endothecium lignification and jasmonic acid biosynthesis.Plant J. 2013 May;74(3):411-22. doi: 10.1111/tpj.12130. Epub 2013 Mar 4. Plant J. 2013. PMID: 23410518
-
PeNAC67-PeKAN2-PeSCL23 and B-class MADS-box transcription factors synergistically regulate the specialization process from petal to lip in Phalaenopsis equestris.Mol Hortic. 2024 Apr 23;4(1):15. doi: 10.1186/s43897-023-00079-8. Mol Hortic. 2024. PMID: 38649966 Free PMC article.
-
How to Evolve a Perianth: A Review of Cadastral Mechanisms for Perianth Identity.Front Plant Sci. 2018 Oct 29;9:1573. doi: 10.3389/fpls.2018.01573. eCollection 2018. Front Plant Sci. 2018. PMID: 30420867 Free PMC article. Review.
-
Floral Induction and Flower Development of Orchids.Front Plant Sci. 2019 Oct 10;10:1258. doi: 10.3389/fpls.2019.01258. eCollection 2019. Front Plant Sci. 2019. PMID: 31649713 Free PMC article. Review.
Cited by
-
Transcriptomic Analysis Suggests Auxin Regulation in Dorsal-Ventral Petal Asymmetry of Wild Progenitor Sinningia speciosa.Int J Mol Sci. 2022 Feb 13;23(4):2073. doi: 10.3390/ijms23042073. Int J Mol Sci. 2022. PMID: 35216188 Free PMC article.
-
Regulatory network for FOREVER YOUNG FLOWER-like genes in regulating Arabidopsis flower senescence and abscission.Commun Biol. 2022 Jul 5;5(1):662. doi: 10.1038/s42003-022-03629-w. Commun Biol. 2022. PMID: 35790878 Free PMC article.
-
TETRASPANIN 8-1 from Phaseolus vulgaris plays a key role during mutualistic interactions.Front Plant Sci. 2023 Jul 3;14:1152493. doi: 10.3389/fpls.2023.1152493. eCollection 2023. Front Plant Sci. 2023. PMID: 37465390 Free PMC article.
-
OAF is a DAF-like gene that controls ovule development in plants.Commun Biol. 2023 May 8;6(1):498. doi: 10.1038/s42003-023-04864-5. Commun Biol. 2023. PMID: 37156904 Free PMC article.
-
Sessile Trichomes Play Major Roles in Prey Digestion and Absorption, While Stalked Trichomes Function in Prey Predation in Byblis guehoi.Int J Mol Sci. 2023 Mar 10;24(6):5305. doi: 10.3390/ijms24065305. Int J Mol Sci. 2023. PMID: 36982381 Free PMC article.
References
-
- Azooz, M. M. , Shaddad, M. A. , & Abdel‐Latef, A. A. (2004). Leaf growth and K+/Na+ ratio as an indication of the salt tolerance of three sorghum cultivars grown under salinity stress and IAA treatment. Acta Agronomica Hungarica, 52, 287–296.
LinkOut - more resources
Full Text Sources