Post-transcriptional gene silencing triggers dispensable DNA methylation in gene body in Arabidopsis
- PMID: 31372641
- PMCID: PMC6753489
- DOI: 10.1093/nar/gkz636
Post-transcriptional gene silencing triggers dispensable DNA methylation in gene body in Arabidopsis
Abstract
Spontaneous post-transcriptional silencing of sense transgenes (S-PTGS) is established in each generation and is accompanied by DNA methylation, but the pathway of PTGS-dependent DNA methylation is unknown and so is its role. Here we show that CHH and CHG methylation coincides spatially and temporally with RDR6-dependent products derived from the central and 3' regions of the coding sequence, and requires the components of the RNA-directed DNA methylation (RdDM) pathway NRPE1, DRD1 and DRM2, but not CLSY1, NRPD1, RDR2 or DCL3, suggesting that RDR6-dependent products, namely long dsRNAs and/or siRNAs, trigger PTGS-dependent DNA methylation. Nevertheless, none of these RdDM components are required to establish S-PTGS or produce a systemic silencing signal. Moreover, preventing de novo DNA methylation in non-silenced transgenic tissues grafted onto homologous silenced tissues does not inhibit the triggering of PTGS. Overall, these data indicate that gene body DNA methylation is a consequence, not a cause, of PTGS, and rule out the hypothesis that a PTGS-associated DNA methylation signal is transmitted independent of a PTGS signal.
© The Author(s) 2019. Published by Oxford University Press on behalf of Nucleic Acids Research.
Figures
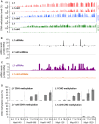
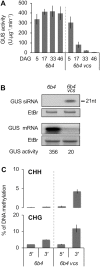
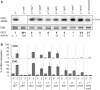
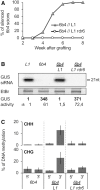
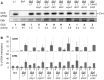
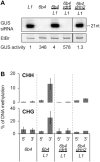
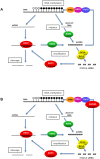
Similar articles
-
NRPD1a and NRPD1b are required to maintain post-transcriptional RNA silencing and RNA-directed DNA methylation in Arabidopsis.Plant J. 2008 Aug;55(4):596-606. doi: 10.1111/j.1365-313X.2008.03525.x. Epub 2008 Apr 22. Plant J. 2008. PMID: 18433438
-
The initiation of epigenetic silencing of active transposable elements is triggered by RDR6 and 21-22 nucleotide small interfering RNAs.Plant Physiol. 2013 May;162(1):116-31. doi: 10.1104/pp.113.216481. Epub 2013 Mar 29. Plant Physiol. 2013. PMID: 23542151 Free PMC article.
-
SHH1, a homeodomain protein required for DNA methylation, as well as RDR2, RDM4, and chromatin remodeling factors, associate with RNA polymerase IV.PLoS Genet. 2011 Jul;7(7):e1002195. doi: 10.1371/journal.pgen.1002195. Epub 2011 Jul 21. PLoS Genet. 2011. PMID: 21811420 Free PMC article.
-
Post-transcriptional gene silencing in plants.J Cell Sci. 2001 Sep;114(Pt 17):3083-91. doi: 10.1242/jcs.114.17.3083. J Cell Sci. 2001. PMID: 11590235 Review.
-
RNA-directed DNA methylation and Pol IVb in Arabidopsis.Cold Spring Harb Symp Quant Biol. 2006;71:449-59. doi: 10.1101/sqb.2006.71.028. Cold Spring Harb Symp Quant Biol. 2006. PMID: 17381327 Review.
Cited by
-
The plant siRNA landscape.Plant Cell. 2024 Jan 30;36(2):246-275. doi: 10.1093/plcell/koad253. Plant Cell. 2024. PMID: 37772967 Free PMC article. Review.
-
Non-coding RNA polymerases that silence transposable elements and reprogram gene expression in plants.Transcription. 2020 Jun-Aug;11(3-4):172-191. doi: 10.1080/21541264.2020.1825906. Epub 2020 Nov 12. Transcription. 2020. PMID: 33180661 Free PMC article. Review.
-
Design of a nanobiosystem with remote photothermal gene silencing in Chlamydomonas reinhardtii to increase lipid accumulation and production.Microb Cell Fact. 2023 Mar 31;22(1):61. doi: 10.1186/s12934-023-02063-9. Microb Cell Fact. 2023. PMID: 37004064 Free PMC article.
-
Virus-Induced Gene Silencing (VIGS): A Powerful Tool for Crop Improvement and Its Advancement towards Epigenetics.Int J Mol Sci. 2023 Mar 15;24(6):5608. doi: 10.3390/ijms24065608. Int J Mol Sci. 2023. PMID: 36982682 Free PMC article. Review.
-
The Insulin Receptor: An Important Target for the Development of Novel Medicines and Pesticides.Int J Mol Sci. 2022 Jul 14;23(14):7793. doi: 10.3390/ijms23147793. Int J Mol Sci. 2022. PMID: 35887136 Free PMC article. Review.
References
-
- Havecker E.R., Wallbridge L.M., Hardcastle T.J., Bush M.S., Kelly K.A., Dunn R.M., Schwach F., Doonan J.H., Baulcombe D.C.. The Arabidopsis RNA-directed DNA methylation argonautes functionally diverge based on their expression and interaction with target loci. Plant Cell. 2010; 22:321–334. - PMC - PubMed
-
- Zhang Z., Liu X., Guo X., Wang X.J., Zhang X.. Arabidopsis AGO3 predominantly recruits 24-nt small RNAs to regulate epigenetic silencing. Nat Plants. 2016; 2:16049. - PubMed
-
- Bologna N.G., Voinnet O.. The diversity, biogenesis, and activities of endogenous silencing small RNAs in Arabidopsis. Annu. Rev. Plant Biol. 2014; 65:473–503. - PubMed
-
- Martinez de Alba A.E., Elvira-Matelot E., Vaucheret H.. Gene silencing in plants: a diversity of pathways. Biochim. Biophys. Acta. 2013; 1829:1300–1308. - PubMed
Publication types
MeSH terms
Substances
LinkOut - more resources
Full Text Sources
Molecular Biology Databases