Ddc2ATRIP promotes Mec1ATR activation at RPA-ssDNA tracts
- PMID: 31369547
- PMCID: PMC6692047
- DOI: 10.1371/journal.pgen.1008294
Ddc2ATRIP promotes Mec1ATR activation at RPA-ssDNA tracts
Abstract
The DNA damage checkpoint response is controlled by the phosphatidylinositol 3-kinase-related kinases (PIKK), including ataxia telangiectasia-mutated (ATM) and ATM and Rad3-related (ATR). ATR forms a complex with its partner ATRIP. In budding yeast, ATR and ATRIP correspond to Mec1 and Ddc2, respectively. ATRIP/Ddc2 interacts with replication protein A-bound single-stranded DNA (RPA-ssDNA) and recruits ATR/Mec1 to sites of DNA damage. Mec1 is stimulated by the canonical activators including Ddc1, Dpb11 and Dna2. We have characterized the ddc2-S4 mutation and shown that Ddc2 not only recruits Mec1 to sites of DNA damage but also stimulates Mec1 kinase activity. However, the underlying mechanism of Ddc2-dependent Mec1 activation remains to be elucidated. Here we show that Ddc2 promotes Mec1 activation independently of Ddc1/Dpb11/Dna2 function in vivo and through ssDNA recognition in vitro. The ddc2-S4 mutation diminishes damage-induced phosphorylation of the checkpoint mediators, Rad9 and Mrc1. Rad9 controls checkpoint throughout the cell-cycle whereas Mrc1 is specifically required for the S-phase checkpoint. Notably, S-phase checkpoint signaling is more defective in ddc2-S4 mutants than in cells where the Mec1 activators (Ddc1/Dpb11 and Dna2) are dysfunctional. To understand a role of Ddc2 in Mec1 activation, we reconstituted an in vitro assay using purified Mec1-Ddc2 complex, RPA and ssDNA. Whereas ssDNA stimulates kinase activity of the Mec1-Ddc2 complex, RPA does not. However, RPA can promote ssDNA-dependent Mec1 activation. Neither ssDNA nor RPA-ssDNA efficiently stimulates the Mec1-Ddc2 complex containing Ddc2-S4 mutant. Together, our data support a model in which Ddc2 promotes Mec1 activation at RPA-ssDNA tracts.
Conflict of interest statement
The authors have declared that no competing interests exist.
Figures
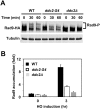
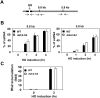
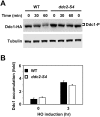
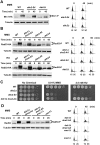
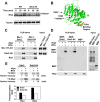
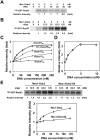
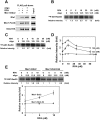
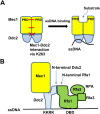
Similar articles
-
Activation of ATR-related protein kinase upon DNA damage recognition.Curr Genet. 2020 Apr;66(2):327-333. doi: 10.1007/s00294-019-01039-w. Epub 2019 Oct 17. Curr Genet. 2020. PMID: 31624858 Free PMC article. Review.
-
Structural Basis of Mec1-Ddc2-RPA Assembly and Activation on Single-Stranded DNA at Sites of Damage.Mol Cell. 2017 Oct 19;68(2):431-445.e5. doi: 10.1016/j.molcel.2017.09.019. Epub 2017 Oct 12. Mol Cell. 2017. PMID: 29033322
-
Ddc2 mediates Mec1 activation through a Ddc1- or Dpb11-independent mechanism.PLoS Genet. 2014 Feb 20;10(2):e1004136. doi: 10.1371/journal.pgen.1004136. eCollection 2014 Feb. PLoS Genet. 2014. PMID: 24586187 Free PMC article.
-
Mec1ATR Autophosphorylation and Ddc2ATRIP Phosphorylation Regulates DNA Damage Checkpoint Signaling.Cell Rep. 2019 Jul 23;28(4):1090-1102.e3. doi: 10.1016/j.celrep.2019.06.068. Cell Rep. 2019. PMID: 31340146 Free PMC article.
-
Cell-cycle-specific activators of the Mec1/ATR checkpoint kinase.Biochem Soc Trans. 2011 Apr;39(2):600-5. doi: 10.1042/BST0390600. Biochem Soc Trans. 2011. PMID: 21428947 Review.
Cited by
-
Activation of ATR-related protein kinase upon DNA damage recognition.Curr Genet. 2020 Apr;66(2):327-333. doi: 10.1007/s00294-019-01039-w. Epub 2019 Oct 17. Curr Genet. 2020. PMID: 31624858 Free PMC article. Review.
-
Resolvases, Dissolvases, and Helicases in Homologous Recombination: Clearing the Road for Chromosome Segregation.Genes (Basel). 2020 Jan 8;11(1):71. doi: 10.3390/genes11010071. Genes (Basel). 2020. PMID: 31936378 Free PMC article. Review.
-
The DNA damage checkpoint: A tale from budding yeast.Front Genet. 2022 Sep 15;13:995163. doi: 10.3389/fgene.2022.995163. eCollection 2022. Front Genet. 2022. PMID: 36186482 Free PMC article. Review.
-
Replication protein-A, RPA, plays a pivotal role in the maintenance of recombination checkpoint in yeast meiosis.Sci Rep. 2024 Apr 25;14(1):9550. doi: 10.1038/s41598-024-60082-x. Sci Rep. 2024. PMID: 38664461 Free PMC article.
-
Novel Cellular Functions of ATR for Therapeutic Targeting: Embryogenesis to Tumorigenesis.Int J Mol Sci. 2023 Jul 20;24(14):11684. doi: 10.3390/ijms241411684. Int J Mol Sci. 2023. PMID: 37511442 Free PMC article. Review.
References
Publication types
MeSH terms
Substances
Grants and funding
LinkOut - more resources
Full Text Sources
Molecular Biology Databases
Research Materials
Miscellaneous