Habituation Learning Is a Widely Affected Mechanism in Drosophila Models of Intellectual Disability and Autism Spectrum Disorders
- PMID: 31272685
- PMCID: PMC7053436
- DOI: 10.1016/j.biopsych.2019.04.029
Habituation Learning Is a Widely Affected Mechanism in Drosophila Models of Intellectual Disability and Autism Spectrum Disorders
Abstract
Background: Although habituation is one of the most ancient and fundamental forms of learning, its regulators and its relevance for human disease are poorly understood.
Methods: We manipulated the orthologs of 286 genes implicated in intellectual disability (ID) with or without comorbid autism spectrum disorder (ASD) specifically in Drosophila neurons, and we tested these models in light-off jump habituation. We dissected neuronal substrates underlying the identified habituation deficits and integrated genotype-phenotype annotations, gene ontologies, and interaction networks to determine the clinical features and molecular processes that are associated with habituation deficits.
Results: We identified >100 genes required for habituation learning. For 93 of these genes, a role in habituation learning was previously unknown. These genes characterize ID disorders with macrocephaly and/or overgrowth and comorbid ASD. Moreover, individuals with ASD from the Simons Simplex Collection carrying damaging de novo mutations in these genes exhibit increased aberrant behaviors associated with inappropriate, stereotypic speech. At the molecular level, ID genes required for normal habituation are enriched in synaptic function and converge on Ras/mitogen-activated protein kinase (Ras/MAPK) signaling. Both increased Ras/MAPK signaling in gamma-aminobutyric acidergic (GABAergic) neurons and decreased Ras/MAPK signaling in cholinergic neurons specifically inhibit the adaptive habituation response.
Conclusions: Our work supports the relevance of habituation learning to ASD, identifies an unprecedented number of novel habituation players, supports an emerging role for inhibitory neurons in habituation, and reveals an opposing, circuit-level-based mechanism for Ras/MAPK signaling. These findings establish habituation as a possible, widely applicable functional readout and target for pharmacologic intervention in ID/ASD.
Keywords: Autism spectrum disorder; Drosophila; GABAergic neurons; Habituation learning; Intellectual disability; Ras/MAPK.
Copyright © 2019 Society of Biological Psychiatry. All rights reserved.
Conflict of interest statement
Financial disclosures:
In the past 3 years, J.C.G. has acted as a consultant to Boehringer Ingelheim GmbH but is not an employee, stock- or share-holder of this company. E.E.E. is on the scientific advisory board (SAB) of DNAnexus, Inc.. Z.A. is a director and shareholder of Aktogen Ltd.. L.A. is a director of Aktogen Ltd.. The commercial light-off jump habituation system was purchased from Aktogen Ltd.. Aktogen Ltd. provided training of the personnel and ~ 150 experiments from the initial screen were performed at Aktogen Ltd. by M.F. and L.A.. All other authors report no biomedical financial interests or potential conflicts of interest.
Figures
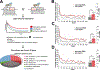
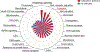
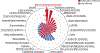
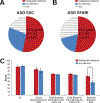
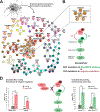
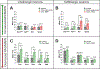
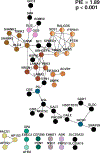
Comment in
-
Dysfunction of Habituation Learning: A Novel Pathogenic Paradigm of Intellectual Disability and Autism Spectrum Disorder.Biol Psychiatry. 2019 Aug 15;86(4):253-254. doi: 10.1016/j.biopsych.2019.06.012. Biol Psychiatry. 2019. PMID: 31370964 No abstract available.
Similar articles
-
Disruption of POGZ Is Associated with Intellectual Disability and Autism Spectrum Disorders.Am J Hum Genet. 2016 Mar 3;98(3):541-552. doi: 10.1016/j.ajhg.2016.02.004. Am J Hum Genet. 2016. PMID: 26942287 Free PMC article.
-
De novo loss-of-function mutations in WAC cause a recognizable intellectual disability syndrome and learning deficits in Drosophila.Eur J Hum Genet. 2016 Aug;24(8):1145-53. doi: 10.1038/ejhg.2015.282. Epub 2016 Jan 13. Eur J Hum Genet. 2016. PMID: 26757981 Free PMC article.
-
Systematic phenomics analysis of autism-associated genes reveals parallel networks underlying reversible impairments in habituation.Proc Natl Acad Sci U S A. 2020 Jan 7;117(1):656-667. doi: 10.1073/pnas.1912049116. Epub 2019 Nov 21. Proc Natl Acad Sci U S A. 2020. PMID: 31754030 Free PMC article.
-
Translating the Role of mTOR- and RAS-Associated Signalopathies in Autism Spectrum Disorder: Models, Mechanisms and Treatment.Genes (Basel). 2021 Oct 30;12(11):1746. doi: 10.3390/genes12111746. Genes (Basel). 2021. PMID: 34828352 Free PMC article. Review.
-
A perspective on molecular signalling dysfunction, its clinical relevance and therapeutics in autism spectrum disorder.Exp Brain Res. 2022 Oct;240(10):2525-2567. doi: 10.1007/s00221-022-06448-x. Epub 2022 Sep 5. Exp Brain Res. 2022. PMID: 36063192 Review.
Cited by
-
Neurofibromin deficiency alters the patterning and prioritization of motor behaviors in a state-dependent manner.bioRxiv [Preprint]. 2024 Aug 9:2024.08.08.607070. doi: 10.1101/2024.08.08.607070. bioRxiv. 2024. PMID: 39149363 Free PMC article. Preprint.
-
Prenatal ethanol exposure impairs sensory processing and habituation to visual stimuli, effects normalized by enrichment of postnatal environmental.Alcohol Clin Exp Res. 2022 May;46(5):891-906. doi: 10.1111/acer.14818. Epub 2022 Apr 18. Alcohol Clin Exp Res. 2022. PMID: 35347730 Free PMC article.
-
Evidence for Prepulse Inhibition of Visually Evoked Motor Response in Drosophila melanogaster.Biology (Basel). 2023 Apr 21;12(4):635. doi: 10.3390/biology12040635. Biology (Basel). 2023. PMID: 37106835 Free PMC article.
-
Choline Transporter regulates olfactory habituation via a neuronal triad of excitatory, inhibitory and mushroom body neurons.PLoS Genet. 2021 Dec 16;17(12):e1009938. doi: 10.1371/journal.pgen.1009938. eCollection 2021 Dec. PLoS Genet. 2021. PMID: 34914708 Free PMC article.
-
The gut-microbiota-brain axis in autism: what Drosophila models can offer?J Neurodev Disord. 2021 Sep 15;13(1):37. doi: 10.1186/s11689-021-09378-x. J Neurodev Disord. 2021. PMID: 34525941 Free PMC article. Review.
References
-
- Peeke H (1973): Habituation: Behavioral studies. (Vol. 1), Academic Press.
-
- Kavšek M (2004): Predicting later IQ from infant visual habituation and dishabituation: A meta-analysis. J Appl Dev Psychol 25.
Publication types
MeSH terms
Grants and funding
LinkOut - more resources
Full Text Sources
Other Literature Sources
Medical
Molecular Biology Databases