Absinthin, an agonist of the bitter taste receptor hTAS2R46, uncovers an ER-to-mitochondria Ca2+-shuttling event
- PMID: 31248983
- PMCID: PMC6699840
- DOI: 10.1074/jbc.RA119.007763
Absinthin, an agonist of the bitter taste receptor hTAS2R46, uncovers an ER-to-mitochondria Ca2+-shuttling event
Abstract
Type 2 taste receptors (TAS2R) are G protein-coupled receptors first described in the gustatory system, but have also been shown to have extraoral localizations, including airway smooth muscle (ASM) cells, in which TAS2R have been reported to induce relaxation. TAS2R46 is an unexplored subtype that responds to its highly specific agonist absinthin. Here, we first demonstrate that, unlike other bitter-taste receptor agonists, absinthin alone (1 μm) in ASM cells does not induce Ca2+ signals but reduces histamine-induced cytosolic Ca2+ increases. To investigate this mechanism, we introduced into ASM cells aequorin-based Ca2+ probes targeted to the cytosol, subplasma membrane domain, or the mitochondrial matrix. We show that absinthin reduces cytosolic histamine-induced Ca2+ rises and simultaneously increases Ca2+ influx into mitochondria. We found that this effect is inhibited by the potent human TAS2R46 (hTAS2R46) antagonist 3β-hydroxydihydrocostunolide and is no longer evident in hTAS2R46-silenced ASM cells, indicating that it is hTAS2R46-dependent. Furthermore, these changes were sensitive to the mitochondrial uncoupler carbonyl cyanide p-(trifluoromethoxy)phenyl-hydrazone (FCCP); the mitochondrial calcium uniporter inhibitor KB-R7943 (carbamimidothioic acid); the cytoskeletal disrupter latrunculin; and an inhibitor of the exchange protein directly activated by cAMP (EPAC), ESI-09. Similarly, the β2 agonist salbutamol also could induce Ca2+ shuttling from cytoplasm to mitochondria, suggesting that this new mechanism might be generalizable. Moreover, forskolin and an EPAC activator mimicked this effect in HeLa cells. Our findings support the hypothesis that plasma membrane receptors can positively regulate mitochondrial Ca2+ uptake, adding a further facet to the ability of cells to encode complex Ca2+ signals.
Keywords: airway smooth muscle; asthma; bitter taste receptors; bronchodilator; calcium; cell signaling; intracellular calcium release; mitochondria; mitochondrial calcium; signal transduction; smooth muscle; type 2 taste receptor (TAS2R).
© 2019 Talmon et al.
Conflict of interest statement
The authors declare that they have no conflicts of interest with the contents of this article
Figures
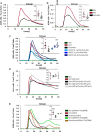
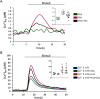
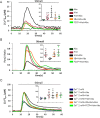
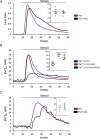
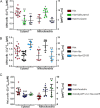
Similar articles
-
Bitter taste receptor agonists alter mitochondrial function and induce autophagy in airway smooth muscle cells.Am J Physiol Lung Cell Mol Physiol. 2017 Jul 1;313(1):L154-L165. doi: 10.1152/ajplung.00106.2017. Epub 2017 Apr 27. Am J Physiol Lung Cell Mol Physiol. 2017. PMID: 28450286 Free PMC article.
-
Antimitogenic effect of bitter taste receptor agonists on airway smooth muscle cells.Am J Physiol Lung Cell Mol Physiol. 2016 Feb 15;310(4):L365-76. doi: 10.1152/ajplung.00373.2015. Epub 2015 Dec 18. Am J Physiol Lung Cell Mol Physiol. 2016. PMID: 26684251 Free PMC article.
-
Molecular mechanism of bitter taste receptor agonist-mediated relaxation of airway smooth muscle.FASEB J. 2024 Jul 31;38(14):e23842. doi: 10.1096/fj.202400452R. FASEB J. 2024. PMID: 39037554
-
Interaction between endoplasmic/sarcoplasmic reticulum stress (ER/SR stress), mitochondrial signaling and Ca(2+) regulation in airway smooth muscle (ASM).Can J Physiol Pharmacol. 2015 Feb;93(2):97-110. doi: 10.1139/cjpp-2014-0361. Epub 2014 Nov 25. Can J Physiol Pharmacol. 2015. PMID: 25506723 Free PMC article. Review.
-
Mitochondrial regulation of cytosolic Ca²⁺ signals in smooth muscle.Pflugers Arch. 2012 Jul;464(1):51-62. doi: 10.1007/s00424-012-1108-9. Epub 2012 May 4. Pflugers Arch. 2012. PMID: 22555917 Review.
Cited by
-
The Complex Journey of the Calcium Regulation Downstream of TAS2R Activation.Cells. 2022 Nov 16;11(22):3638. doi: 10.3390/cells11223638. Cells. 2022. PMID: 36429066 Free PMC article. Review.
-
Bitter Taste Receptor 46 (hTAS2R46) Protects Monocytes/Macrophages from Oxidative Stress.Int J Mol Sci. 2024 Jul 3;25(13):7325. doi: 10.3390/ijms25137325. Int J Mol Sci. 2024. PMID: 39000432 Free PMC article.
-
Phenanthroline relaxes uterine contractions induced by diverse contractile agents by decreasing cytosolic calcium concentration.Eur J Pharmacol. 2024 Apr 5;968:176343. doi: 10.1016/j.ejphar.2024.176343. Epub 2024 Jan 26. Eur J Pharmacol. 2024. PMID: 38281680
-
Mast cells in asthma: Here I am, stuck in the middle with you.Eur Respir J. 2020 Jul 2;56(1):2001337. doi: 10.1183/13993003.01337-2020. Print 2020 Jul. Eur Respir J. 2020. PMID: 32616549 Free PMC article. No abstract available.
-
TAS2R16 Activation Suppresses LPS-Induced Cytokine Expression in Human Gingival Fibroblasts.Front Immunol. 2021 Dec 15;12:726546. doi: 10.3389/fimmu.2021.726546. eCollection 2021. Front Immunol. 2021. PMID: 34975834 Free PMC article.
References
Publication types
MeSH terms
Substances
LinkOut - more resources
Full Text Sources
Molecular Biology Databases
Miscellaneous