Recent Developments of Supramolecular Metal-based Structures for Applications in Cancer Therapy and Imaging
- PMID: 31244947
- PMCID: PMC6567972
- DOI: 10.7150/thno.31828
Recent Developments of Supramolecular Metal-based Structures for Applications in Cancer Therapy and Imaging
Abstract
The biomedical application of discrete supramolecular metal-based structures, including supramolecular coordination complexes (SCCs), is still an emergent field of study. However, pioneering studies over the last 10 years demonstrated the potential of these supramolecular compounds as novel anticancer drugs, endowed with different mechanisms of action compared to classical small-molecules, often related to their peculiar molecular recognition properties. In addition, the robustness and modular composition of supramolecular metal-based structures allows for an incorporation of different functionalities in the same system to enable imaging in cells via different modalities, but also active tumor targeting and stimuli-responsiveness. Although most of the studies reported so far exploit these systems for therapy, supramolecular metal-based structures may also constitute ideal scaffolds to develop multimodal theranostic agents. Of note, the host-guest chemistry of 3D self-assembled supramolecular structures - within the metallacages family - can also be exploited to design novel drug delivery systems for anticancer chemotherapeutics. In this review, we aim at summarizing the pivotal concepts in this fascinating research area, starting with the main design principles and illustrating representative examples while providing a critical discussion of the state-of-the-art. A section is also included on supramolecular organometallic complexes (SOCs) whereby the (organic) linker is forming the organometallic bond to the metal node, whose biological applications are still to be explored. Certainly, the myriad of possible supramolecular metal-based structures and their almost limitless modularity and tunability suggests that the biomedical applications of such complex chemical entities will continue along this already promising path.
Keywords: supramolecular metal-based complexes - metallacages - cancer - drug delivery - theranostics..
Conflict of interest statement
Competing Interests: The authors have declared that no competing interest exists.
Figures
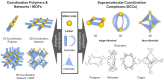
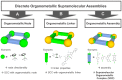
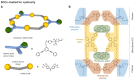
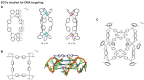
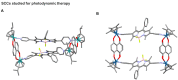
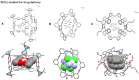
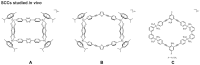
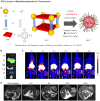
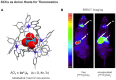
Similar articles
-
Metal-Organic Framework Nanoparticle-Based Biomineralization: A New Strategy toward Cancer Treatment.Theranostics. 2019 May 18;9(11):3134-3149. doi: 10.7150/thno.33539. eCollection 2019. Theranostics. 2019. PMID: 31244946 Free PMC article. Review.
-
Polyphenol-Based Particles for Theranostics.Theranostics. 2019 May 18;9(11):3170-3190. doi: 10.7150/thno.31847. eCollection 2019. Theranostics. 2019. PMID: 31244948 Free PMC article. Review.
-
Bioengineering of Metal-organic Frameworks for Nanomedicine.Theranostics. 2019 May 18;9(11):3122-3133. doi: 10.7150/thno.31918. eCollection 2019. Theranostics. 2019. PMID: 31244945 Free PMC article. Review.
-
Biomedical and biochemical applications of self-assembled metallacycles and metallacages.Acc Chem Res. 2013 Nov 19;46(11):2464-74. doi: 10.1021/ar400010v. Epub 2013 Jun 20. Acc Chem Res. 2013. PMID: 23786636 Free PMC article.
-
DNA-supramolecule conjugates in theranostics.Theranostics. 2019 May 18;9(11):3262-3279. doi: 10.7150/thno.31885. eCollection 2019. Theranostics. 2019. PMID: 31244953 Free PMC article. Review.
Cited by
-
Exploiting reduced-symmetry ligands with pyridyl and imidazole donors to construct a second-generation stimuli-responsive heterobimetallic [PdPtL4]4+ cage.Chem Sci. 2023 Jul 14;14(32):8615-8623. doi: 10.1039/d3sc01354e. eCollection 2023 Aug 16. Chem Sci. 2023. PMID: 37592996 Free PMC article.
-
Selective formation of Pt12L24 nanospheres by ligand design.Chem Sci. 2021 Apr 28;12(22):7696-7705. doi: 10.1039/d1sc01295a. Chem Sci. 2021. PMID: 34168821 Free PMC article.
-
Pillararene-Based Supramolecular Polymers for Cancer Therapy.Molecules. 2023 Feb 3;28(3):1470. doi: 10.3390/molecules28031470. Molecules. 2023. PMID: 36771136 Free PMC article. Review.
-
Metals in Cancer Research: Beyond Platinum Metallodrugs.ACS Cent Sci. 2024 Feb 7;10(2):242-250. doi: 10.1021/acscentsci.3c01340. eCollection 2024 Feb 28. ACS Cent Sci. 2024. PMID: 38435529 Free PMC article. Review.
-
Comparing organic and metallo-organic hydrazone molecular cages as potential carriers for doxorubicin delivery.Chem Sci. 2024 Jun 7;15(26):10010-10017. doi: 10.1039/d4sc02294g. eCollection 2024 Jul 3. Chem Sci. 2024. PMID: 38966373 Free PMC article.
References
-
- James SL. Metal-organic frameworks. Chem Soc Rev. 2003;32:276–288. - PubMed
-
- Zhou HC, Long JR, Yaghi OM. Introduction to metal-organic frameworks. Chem Rev. 2012;112:673–674. - PubMed
-
- Cook TR, Stang PJ. Recent developments in the preparation and chemistry of metallacycles and metallacages via coordination. Chem Rev. 2015;115:7001–7045. - PubMed
Publication types
MeSH terms
Substances
LinkOut - more resources
Full Text Sources