Human Cytomegalovirus Disruption of Calcium Signaling in Neural Progenitor Cells and Organoids
- PMID: 31217241
- PMCID: PMC6694809
- DOI: 10.1128/JVI.00954-19
Human Cytomegalovirus Disruption of Calcium Signaling in Neural Progenitor Cells and Organoids
Abstract
The herpesvirus human cytomegalovirus (HCMV) is a leading cause of congenital birth defects. Infection can result in infants born with a variety of symptoms, including hepatosplenomegaly, microcephaly, and developmental disabilities. Microcephaly is associated with disruptions in the neural progenitor cell (NPC) population. Here, we defined the impact of HCMV infection on neural tissue development and calcium regulation, a critical activity in neural development. Regulation of intracellular calcium involves purinergic receptors and voltage-gated calcium channels (VGCC). HCMV infection compromised the ability of both pathways in NPCs as well as fibroblasts to respond to stimulation. We observed significant drops in basal calcium levels in infected NPCs which were accompanied by loss in VGCC activity and purinergic receptor responses. However, uninfected cells in the population retained responsiveness. Addition of the HCMV inhibitor maribavir reduced viral spread but failed to restore activity in infected cells. To study neural development, we infected three-dimensional cortical organoids with HCMV. Infection spread to a subset of cells over time and disrupted organoid structure, with alterations in developmental and neural layering markers. Organoid-derived infected neurons and astrocytes were unable to respond to stimulation whereas uninfected cells retained nearly normal responses. Maribavir partially restored structural features, including neural rosette formation, and dampened the impact of infection on neural cellular function. Using a tissue model system, we have demonstrated that HCMV alters cortical neural layering and disrupts calcium regulation in infected cells.IMPORTANCE Human cytomegalovirus (HCMV) replicates in several cell types throughout the body, causing disease in the absence of an effective immune response. Studies on HCMV require cultured human cells and tissues due to species specificity. In these studies, we investigated the impact of infection on developing three-dimensional cortical organoid tissues, with specific emphasis on cell-type-dependent calcium signaling. Calcium signaling is an essential function during neural differentiation and cortical development. We observed that HCMV infects and spreads within these tissues, ultimately disrupting cortical structure. Infected cells exhibited depleted calcium stores and loss of ATP- and KCl-stimulated calcium signaling while uninfected cells in the population maintained nearly normal responses. Some protection was provided by the viral inhibitor maribavir. Overall, our studies provide new insights into the impact of HCMV on cortical tissue development and function.
Keywords: astrocytes; calcium signaling; cytomegalovirus; maribavir; microcephaly; neural stem cells; neurons; organoids.
Copyright © 2019 American Society for Microbiology.
Figures
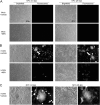
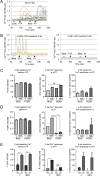
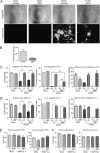
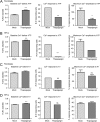
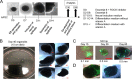
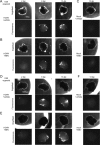
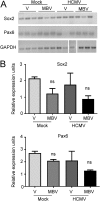
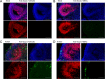
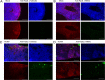
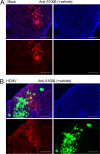
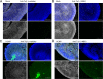
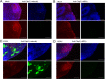
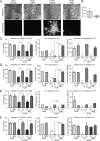
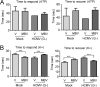
Similar articles
-
Human Cytomegalovirus Compromises Development of Cerebral Organoids.J Virol. 2019 Aug 13;93(17):e00957-19. doi: 10.1128/JVI.00957-19. Print 2019 Sep 1. J Virol. 2019. PMID: 31217239 Free PMC article.
-
Nitric Oxide Attenuates Human Cytomegalovirus Infection yet Disrupts Neural Cell Differentiation and Tissue Organization.J Virol. 2022 Jul 27;96(14):e0012622. doi: 10.1128/jvi.00126-22. Epub 2022 Jul 7. J Virol. 2022. PMID: 35862705 Free PMC article.
-
Impact of a cytomegalovirus kinase inhibitor on infection and neuronal progenitor cell differentiation.Antiviral Res. 2016 May;129:67-73. doi: 10.1016/j.antiviral.2016.02.007. Epub 2016 Feb 11. Antiviral Res. 2016. PMID: 26875788 Free PMC article.
-
Clinical development of letermovir and maribavir: Overview of human cytomegalovirus drug resistance.Antiviral Res. 2019 Mar;163:91-105. doi: 10.1016/j.antiviral.2019.01.011. Epub 2019 Jan 25. Antiviral Res. 2019. PMID: 30690043 Review.
-
[Interrelationship between human cytomegalovirus infection and chemokine].Nihon Rinsho. 1998 Jan;56(1):69-74. Nihon Rinsho. 1998. PMID: 9465667 Review. Japanese.
Cited by
-
Brain organoids for addressing COVID-19 challenge.Front Neurosci. 2022 Nov 29;16:1055601. doi: 10.3389/fnins.2022.1055601. eCollection 2022. Front Neurosci. 2022. PMID: 36523428 Free PMC article. Review.
-
iPSC-derived three-dimensional brain organoid models and neurotropic viral infections.J Neurovirol. 2023 Apr;29(2):121-134. doi: 10.1007/s13365-023-01133-3. Epub 2023 Apr 25. J Neurovirol. 2023. PMID: 37097597 Free PMC article. Review.
-
HCMV Infection Reduces Nidogen-1 Expression, Contributing to Impaired Neural Rosette Development in Brain Organoids.J Virol. 2023 May 31;97(5):e0171822. doi: 10.1128/jvi.01718-22. Epub 2023 May 1. J Virol. 2023. PMID: 37125912 Free PMC article.
-
Interplay Between Calcium and AMPK Signaling in Human Cytomegalovirus Infection.Front Cell Infect Microbiol. 2020 Jul 29;10:384. doi: 10.3389/fcimb.2020.00384. eCollection 2020. Front Cell Infect Microbiol. 2020. PMID: 32850483 Free PMC article. Review.
-
Modeling SARS-CoV-2 infection in individuals with opioid use disorder with brain organoids.J Tissue Eng. 2021 Feb 26;12:2041731420985299. doi: 10.1177/2041731420985299. eCollection 2021 Jan-Dec. J Tissue Eng. 2021. PMID: 33738089 Free PMC article. Review.
References
-
- Mocarski ES, Shenk T, Griffiths PD, Pass RF. 2013. Cytomegaloviruses, p 1960–2014. In Knipe DM, Howley PM, Cohen JI, Griffin DE, Lamb RA, Martin MA, Rancaniello VR, Roizman B (ed), Fields virology, 6 ed, vol 2 Lippincott Williams & Wilkins, Philadelphia, PA.
-
- Weekes MP, Tomasec P, Huttlin EL, Fielding CA, Nusinow D, Stanton RJ, Wang EC, Aicheler R, Murrell I, Wilkinson GW, Lehner PJ, Gygi SP. 2014. Quantitative temporal viromics: an approach to investigate host-pathogen interaction. Cell 157:1460–1472. doi:10.1016/j.cell.2014.04.028. - DOI - PMC - PubMed
Publication types
MeSH terms
Substances
Grants and funding
LinkOut - more resources
Full Text Sources
Medical
Research Materials