Tales of tails in transporters
- PMID: 31213137
- PMCID: PMC6597760
- DOI: 10.1098/rsob.190083
Tales of tails in transporters
Abstract
Cell nutrition, detoxification, signalling, homeostasis and response to drugs, processes related to cell growth, differentiation and survival are all mediated by plasma membrane (PM) proteins called transporters. Despite their distinct fine structures, mechanism of function, energetic requirements, kinetics and substrate specificities, all transporters are characterized by a main hydrophobic body embedded in the PM as a series of tightly packed, often intertwined, α-helices that traverse the lipid bilayer in a zigzag mode, connected with intracellular or extracellular loops and hydrophilic N- and C-termini. Whereas longstanding genetic, biochemical and biophysical evidence suggests that specific transmembrane segments, and also their connecting loops, are responsible for substrate recognition and transport dynamics, emerging evidence also reveals the functional importance of transporter N- and C-termini, in respect to transport catalysis, substrate specificity, subcellular expression, stability and signalling. This review highlights selected prototypic examples of transporters in which their termini play important roles in their functioning.
Keywords: fluorescent microscopy; model fungi; molecular dynamics; mutational analysis; phylogeny; transporter structure.
Conflict of interest statement
We declare we have no competing interests.
Figures
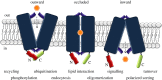
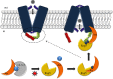
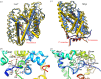
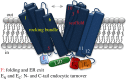
Similar articles
-
From membrane to molecule to the third amino acid from the left with a membrane transport protein.Q Rev Biophys. 1997 Nov;30(4):333-64. doi: 10.1017/s0033583597003387. Q Rev Biophys. 1997. PMID: 9634651 Review.
-
Cytosolic N- and C-Termini of the Aspergillus nidulans FurE Transporter Contain Distinct Elements that Regulate by Long-Range Effects Function and Specificity.J Mol Biol. 2019 Sep 6;431(19):3827-3844. doi: 10.1016/j.jmb.2019.07.013. Epub 2019 Jul 12. J Mol Biol. 2019. PMID: 31306663
-
The Structure of a Sugar Transporter of the Glucose EIIC Superfamily Provides Insight into the Elevator Mechanism of Membrane Transport.Structure. 2016 Jun 7;24(6):956-64. doi: 10.1016/j.str.2016.04.003. Epub 2016 May 5. Structure. 2016. PMID: 27161976 Free PMC article.
-
Structural insights into functional lipid-protein interactions in secondary transporters.Biochim Biophys Acta. 2015 Mar;1850(3):476-87. doi: 10.1016/j.bbagen.2014.05.010. Epub 2014 May 20. Biochim Biophys Acta. 2015. PMID: 24859688 Review.
-
Prediction of membrane transport proteins and their substrate specificities using primary sequence information.PLoS One. 2014 Jun 26;9(6):e100278. doi: 10.1371/journal.pone.0100278. eCollection 2014. PLoS One. 2014. PMID: 24968309 Free PMC article.
Cited by
-
Studies on sugar transporter CRT1 reveal new characteristics that are critical for cellulase induction in Trichoderma reesei.Biotechnol Biofuels. 2020 Sep 14;13:158. doi: 10.1186/s13068-020-01797-7. eCollection 2020. Biotechnol Biofuels. 2020. PMID: 32944074 Free PMC article.
-
A guide to plasma membrane solute carrier proteins.FEBS J. 2021 May;288(9):2784-2835. doi: 10.1111/febs.15531. Epub 2020 Sep 18. FEBS J. 2021. PMID: 32810346 Free PMC article. Review.
-
α-Synuclein and Mitochondria: Probing the Dynamics of Disordered Membrane-protein Regions Using Solid-State Nuclear Magnetic Resonance.JACS Au. 2024 May 28;4(6):2372-2380. doi: 10.1021/jacsau.4c00323. eCollection 2024 Jun 24. JACS Au. 2024. PMID: 38938811 Free PMC article.
-
SLC38A10 Regulate Glutamate Homeostasis and Modulate the AKT/TSC2/mTOR Pathway in Mouse Primary Cortex Cells.Front Cell Dev Biol. 2022 Apr 5;10:854397. doi: 10.3389/fcell.2022.854397. eCollection 2022. Front Cell Dev Biol. 2022. PMID: 35450293 Free PMC article.
-
The Poly-Glutamate Motif of GmMATE4 Regulates Its Isoflavone Transport Activity.Membranes (Basel). 2022 Feb 10;12(2):206. doi: 10.3390/membranes12020206. Membranes (Basel). 2022. PMID: 35207127 Free PMC article.
References
Publication types
MeSH terms
Substances
LinkOut - more resources
Full Text Sources