Nitric Oxide and Hydrogen Sulfide Regulation of Ischemic Vascular Growth and Remodeling
- PMID: 31187898
- PMCID: PMC6938731
- DOI: 10.1002/cphy.c180026
Nitric Oxide and Hydrogen Sulfide Regulation of Ischemic Vascular Growth and Remodeling
Abstract
Ischemic vascular remodeling occurs in response to stenosis or arterial occlusion leading to a change in blood flow and tissue perfusion. Altered blood flow elicits a cascade of molecular and cellular physiological responses leading to vascular remodeling of the macro- and micro-circulation. Although cellular mechanisms of vascular remodeling such as arteriogenesis and angiogenesis have been studied, therapeutic approaches in these areas have had limited success due to the complexity and heterogeneous constellation of molecular signaling events regulating these processes. Understanding central molecular players of vascular remodeling should lead to a deeper understanding of this response and aid in the development of novel therapeutic strategies. Hydrogen sulfide (H2 S) and nitric oxide (NO) are gaseous signaling molecules that are critically involved in regulating fundamental biochemical and molecular responses necessary for vascular growth and remodeling. This review examines how NO and H2 S regulate pathophysiological mechanisms of angiogenesis and arteriogenesis, along with important chemical and experimental considerations revealed thus far. The importance of NO and H2 S bioavailability, their synthesis enzymes and cofactors, and genetic variations associated with cardiovascular risk factors suggest that they serve as pivotal regulators of vascular remodeling responses. © 2019 American Physiological Society. Compr Physiol 9:1213-1247, 2019.
Copyright © 2019 American Physiological Society. All rights reserved.
Figures
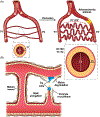
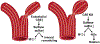
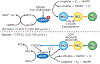
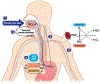
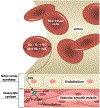
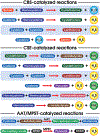
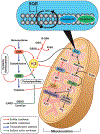
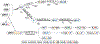
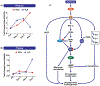
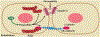
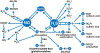
Similar articles
-
Nitric Oxide and Hydrogen Sulfide Regulation of Ischemic Vascular Remodeling.Microcirculation. 2016 Feb;23(2):134-45. doi: 10.1111/micc.12248. Microcirculation. 2016. PMID: 26381654 Free PMC article. Review.
-
Remodeling of Cerebral Microcirculation after Ischemia-Reperfusion.J Vasc Res. 2015;52(1):22-31. doi: 10.1159/000381096. Epub 2015 Apr 21. J Vasc Res. 2015. PMID: 25896412 Review.
-
Cystathionine γ-Lyase Modulates Flow-Dependent Vascular Remodeling.Arterioscler Thromb Vasc Biol. 2018 Sep;38(9):2126-2136. doi: 10.1161/ATVBAHA.118.311402. Arterioscler Thromb Vasc Biol. 2018. PMID: 30002061 Free PMC article.
-
Regulation and role of endogenously produced hydrogen sulfide in angiogenesis.Pharmacol Res. 2016 Nov;113(Pt A):175-185. doi: 10.1016/j.phrs.2016.08.026. Epub 2016 Aug 26. Pharmacol Res. 2016. PMID: 27569706 Free PMC article. Review.
-
Revealing on hydrogen sulfide and nitric oxide signals co-ordination for plant growth under stress conditions.Physiol Plant. 2020 Feb;168(2):301-317. doi: 10.1111/ppl.13002. Epub 2019 Jul 2. Physiol Plant. 2020. PMID: 31264712 Review.
Cited by
-
Association Between Thyroid Parameters and Subclinical Atherosclerosis in Hospitalised Euthyroid Patients with Type 2 Diabetes Mellitus.Diabetes Metab Syndr Obes. 2023 Oct 12;16:3163-3171. doi: 10.2147/DMSO.S429941. eCollection 2023. Diabetes Metab Syndr Obes. 2023. PMID: 37849978 Free PMC article.
-
The lncRNA Punisher Regulates Apoptosis and Mitochondrial Homeostasis of Vascular Smooth Muscle Cells via Targeting miR-664a-5p and OPA1.Oxid Med Cell Longev. 2022 May 25;2022:5477024. doi: 10.1155/2022/5477024. eCollection 2022. Oxid Med Cell Longev. 2022. PMID: 35663194 Free PMC article.
-
Inhibition of CCL28/CCR10-Mediated eNOS Downregulation Improves Skin Wound Healing in the Obesity-Induced Mouse Model of Type 2 Diabetes.Diabetes. 2022 Oct 1;71(10):2166-2180. doi: 10.2337/db21-1108. Diabetes. 2022. PMID: 35899992 Free PMC article.
-
Nitric Oxide Signaling for Aerial Mycelium Formation in Streptomyces coelicolor A3(2) M145.Appl Environ Microbiol. 2022 Dec 13;88(23):e0122222. doi: 10.1128/aem.01222-22. Epub 2022 Nov 10. Appl Environ Microbiol. 2022. PMID: 36354316 Free PMC article.
-
Role of hydrogen sulphide in physiological and pathological angiogenesis.Cell Prolif. 2023 Mar;56(3):e13374. doi: 10.1111/cpr.13374. Epub 2022 Dec 7. Cell Prolif. 2023. PMID: 36478328 Free PMC article. Review.
References
-
- Achan V, Broadhead M, Malaki M, Whitley G, Leiper J, MacAllister R, Vallance P. Asymmetric dimethylarginine causes hypertension and cardiac dysfunction in humans and is actively metabolized by dimethylarginine dimethylaminohydrolase. Arterioscler Thromb Vasc Biol 23: 1455–1459, 2003. - PubMed
-
- Aicher A, Heeschen C, Mildner-Rihm C, Urbich C, Ihling C, Technau-Ihling K, Zeiher AM, Dimmeler S. Essential role of endothelial nitric oxide synthase for mobilization of stem and progenitor cells. Nat Med 9: 1370–1376, 2003. - PubMed
-
- Aras O, Hanson NQ, Yang F, Tsai MY. Influence of 699C→T and 1080C→T polymorphisms of the cystathionine beta-synthase gene on plasma homocysteine levels. Clin Genet 58: 455–459, 2000. - PubMed
Publication types
MeSH terms
Substances
Grants and funding
LinkOut - more resources
Full Text Sources