Establishment of porcine and human expanded potential stem cells
- PMID: 31160711
- PMCID: PMC7035105
- DOI: 10.1038/s41556-019-0333-2
Establishment of porcine and human expanded potential stem cells
Abstract
We recently derived mouse expanded potential stem cells (EPSCs) from individual blastomeres by inhibiting the critical molecular pathways that predispose their differentiation. EPSCs had enriched molecular signatures of blastomeres and possessed developmental potency for all embryonic and extra-embryonic cell lineages. Here, we report the derivation of porcine EPSCs, which express key pluripotency genes, are genetically stable, permit genome editing, differentiate to derivatives of the three germ layers in chimeras and produce primordial germ cell-like cells in vitro. Under similar conditions, human embryonic stem cells and induced pluripotent stem cells can be converted, or somatic cells directly reprogrammed, to EPSCs that display the molecular and functional attributes reminiscent of porcine EPSCs. Importantly, trophoblast stem-cell-like cells can be generated from both human and porcine EPSCs. Our pathway-inhibition paradigm thus opens an avenue for generating mammalian pluripotent stem cells, and EPSCs present a unique cellular platform for translational research in biotechnology and regenerative medicine.
Figures
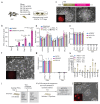
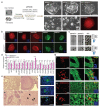
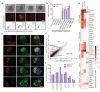
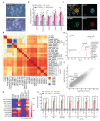
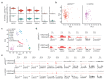
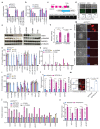
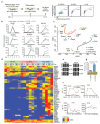
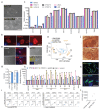
Comment in
-
A boost towards totipotency for stem cells.Nat Cell Biol. 2019 Jun;21(6):671-673. doi: 10.1038/s41556-019-0340-3. Nat Cell Biol. 2019. PMID: 31160707 No abstract available.
-
Stem cells with potential.Nat Methods. 2019 Jul;16(7):578. doi: 10.1038/s41592-019-0487-7. Nat Methods. 2019. PMID: 31249416 No abstract available.
-
Modeling the Placenta with Stem Cells.N Engl J Med. 2019 Oct 24;381(17):1681-1683. doi: 10.1056/NEJMcibr1907773. N Engl J Med. 2019. PMID: 31644851 No abstract available.
Similar articles
-
In vitro establishment of expanded-potential stem cells from mouse pre-implantation embryos or embryonic stem cells.Nat Protoc. 2019 Feb;14(2):350-378. doi: 10.1038/s41596-018-0096-4. Nat Protoc. 2019. PMID: 30617351
-
Capturing Pluripotency and Beyond.Cells. 2021 Dec 16;10(12):3558. doi: 10.3390/cells10123558. Cells. 2021. PMID: 34944066 Free PMC article. Review.
-
Apoptosis in Porcine Pluripotent Cells: From ICM to iPSCs.Int J Mol Sci. 2016 Sep 12;17(9):1533. doi: 10.3390/ijms17091533. Int J Mol Sci. 2016. PMID: 27626414 Free PMC article. Review.
-
An optimized culture system for efficient derivation of porcine expanded potential stem cells from preimplantation embryos and by reprogramming somatic cells.Nat Protoc. 2024 Jun;19(6):1710-1749. doi: 10.1038/s41596-024-00958-4. Epub 2024 Mar 20. Nat Protoc. 2024. PMID: 38509352 Review.
-
Primed pluripotent cell lines derived from various embryonic origins and somatic cells in pig.PLoS One. 2013;8(1):e52481. doi: 10.1371/journal.pone.0052481. Epub 2013 Jan 11. PLoS One. 2013. PMID: 23326334 Free PMC article.
Cited by
-
Modeling Paratuberculosis in Laboratory Animals, Cells, or Tissues: A Focus on Their Applications for Pathogenesis, Diagnosis, Vaccines, and Therapy Studies.Animals (Basel). 2023 Nov 17;13(22):3553. doi: 10.3390/ani13223553. Animals (Basel). 2023. PMID: 38003170 Free PMC article. Review.
-
Human early syncytiotrophoblasts are highly susceptible to SARS-CoV-2 infection.Cell Rep Med. 2022 Dec 20;3(12):100849. doi: 10.1016/j.xcrm.2022.100849. Epub 2022 Nov 17. Cell Rep Med. 2022. PMID: 36495872 Free PMC article.
-
Generation of Pericytic-Vascular Progenitors from Tankyrase/PARP-Inhibitor-Regulated Naïve (TIRN) Human Pluripotent Stem Cells.Methods Mol Biol. 2022;2416:133-156. doi: 10.1007/978-1-0716-1908-7_10. Methods Mol Biol. 2022. PMID: 34870835 Free PMC article.
-
Derivation of Porcine Extra-Embryonic Endoderm Cell Lines Reveals Distinct Signaling Pathway and Multipotency States.Int J Mol Sci. 2021 Nov 29;22(23):12918. doi: 10.3390/ijms222312918. Int J Mol Sci. 2021. PMID: 34884722 Free PMC article.
-
Single-cell 3D genome structure reveals distinct human pluripotent states.Genome Biol. 2024 May 13;25(1):122. doi: 10.1186/s13059-024-03268-w. Genome Biol. 2024. PMID: 38741214 Free PMC article.
References
Publication types
MeSH terms
Grants and funding
LinkOut - more resources
Full Text Sources
Other Literature Sources
Research Materials