Structural and Evolutionary Insights Point to Allosteric Regulation of TRP Ion Channels
- PMID: 31149807
- PMCID: PMC8628317
- DOI: 10.1021/acs.accounts.9b00075
Structural and Evolutionary Insights Point to Allosteric Regulation of TRP Ion Channels
Abstract
The familiar pungent taste of spicy food, the refreshing taste of mint, and many other physiological phenomena are mediated by transient receptor potential (TRP) ion channels. TRP channels are a superfamily of ion channels that are sensitive to diverse chemical and physical stimuli and play diverse roles in biology. In addition to chemical regulation, some family members also sense common physical stimuli, such as temperature or pressure. Since their discovery and cloning in the 1990s and 2000s, understanding the molecular mechanisms governing TRP channel function and polymodal regulation has been a consistent but challenging goal. Until recently, a general lack of high-resolution TRP channel structures had significantly limited a molecular understanding of their function. In the past few years, a flood of TRP channel structures have been released, made possible primarily by advances in cryo-electron microscopy (cryo-EM). The boon of many structures has unleashed unparalleled insight into TRP channel architecture. Substantive comparative studies between TRP structures provide snapshots of distinct states such as ligand-free, stabilized by chemical agonists, or antagonists, partially illuminating how a given channel opens and closes. However, the now ∼75 TRP channel structures have ushered in surprising outcomes, including a lack of an apparent general mechanism underlying channel opening and closing among family members. Similarly, the structures reveal a surprising diversity in which chemical ligands bind TRP channels. Several TRP channels are activated by temperature changes in addition to ligand binding. Unraveling mechanisms of thermosensation has proven an elusive challenge to the field. Although some studies point to thermosensitive domains in the transmembrane region of the channels, results have sometimes been contradictory and difficult to interpret; in some cases, a domain that proves essential for thermal sensitivity in one context can be entirely removed from the channel without affecting thermosensation in another context. These results are not amenable to simple interpretations and point to allosteric networks of regulation within the channel structure. TRP channels have evolved to be fine-tuned for the needs of a species in its environmental niche, a fact that has been both a benefit and burden in unlocking their molecular features. Functional evolutionary divergence has presented challenges for studying TRP channels, as orthologs from different species can give conflicting experimental results. However, this diversity can also be examined comparatively to decipher the basis for functional differences. As with structural biology, untangling the similarities and differences resulting from evolutionary pressure between species has been a rich source of data guiding the field. This Account will contextualize the existing biochemical and functional data with an eye to evolutionary data and couple these insights with emerging structural biology to better understand the molecular mechanisms behind chemical and physical regulation of TRP channels.
Figures
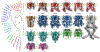
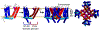
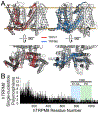
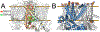
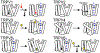
Similar articles
-
Determining the Crystal Structure of TRPV6.In: Kozak JA, Putney JW Jr, editors. Calcium Entry Channels in Non-Excitable Cells. Boca Raton (FL): CRC Press/Taylor & Francis; 2018. Chapter 14. In: Kozak JA, Putney JW Jr, editors. Calcium Entry Channels in Non-Excitable Cells. Boca Raton (FL): CRC Press/Taylor & Francis; 2018. Chapter 14. PMID: 30299652 Free Books & Documents. Review.
-
Structural biology of thermoTRPV channels.Cell Calcium. 2019 Dec;84:102106. doi: 10.1016/j.ceca.2019.102106. Epub 2019 Nov 1. Cell Calcium. 2019. PMID: 31726322 Free PMC article. Review.
-
Allosterism and Structure in Thermally Activated Transient Receptor Potential Channels.Annu Rev Biophys. 2016 Jul 5;45:371-98. doi: 10.1146/annurev-biophys-062215-011034. Epub 2016 May 23. Annu Rev Biophys. 2016. PMID: 27297398 Review.
-
Structural mechanisms of transient receptor potential ion channels.J Gen Physiol. 2020 Mar 2;152(3):e201811998. doi: 10.1085/jgp.201811998. J Gen Physiol. 2020. PMID: 31972006 Free PMC article. Review.
-
Structural Pharmacology of TRP Channels.J Mol Biol. 2021 Aug 20;433(17):166914. doi: 10.1016/j.jmb.2021.166914. Epub 2021 Mar 5. J Mol Biol. 2021. PMID: 33676926 Free PMC article. Review.
Cited by
-
Irritant-evoked activation and calcium modulation of the TRPA1 receptor.Nature. 2020 Sep;585(7823):141-145. doi: 10.1038/s41586-020-2480-9. Epub 2020 Jul 8. Nature. 2020. PMID: 32641835 Free PMC article.
-
Membrane Hormone Receptors and Their Signaling Pathways as Targets for Endocrine Disruptors.J Xenobiot. 2022 Mar 25;12(2):64-73. doi: 10.3390/jox12020007. J Xenobiot. 2022. PMID: 35466213 Free PMC article. Review.
-
Thermosensing ability of TRPC5: current knowledge and unsettled questions.J Physiol Sci. 2024 Oct 3;74(1):50. doi: 10.1186/s12576-024-00942-3. J Physiol Sci. 2024. PMID: 39363236 Free PMC article. Review.
-
A cooperative knock-on mechanism underpins Ca2+-selective cation permeation in TRPV channels.J Gen Physiol. 2023 May 1;155(5):e202213226. doi: 10.1085/jgp.202213226. Epub 2023 Mar 21. J Gen Physiol. 2023. PMID: 36943243 Free PMC article.
-
Simulation and Machine Learning Methods for Ion-Channel Structure Determination, Mechanistic Studies and Drug Design.Front Pharmacol. 2022 Jun 28;13:939555. doi: 10.3389/fphar.2022.939555. eCollection 2022. Front Pharmacol. 2022. PMID: 35837274 Free PMC article. Review.
References
-
- Ciardo MG; Ferrer-Montiel A Lipids as central modulators of sensory TRP channels. Biochim Biophys Acta Biomembr 2017, 1859, 1615–1628. - PubMed
-
- Numazaki M; Tominaga T; Toyooka H; Tominaga M Direct phosphorylation of capsaicin receptor VR1 by protein kinase Cepsilon and identification of two target serine residues. J Biol Chem 2002, 277, 13375–13378. - PubMed
Publication types
MeSH terms
Substances
Grants and funding
LinkOut - more resources
Full Text Sources
Research Materials
Miscellaneous