Phosphatidylinositol 4,5-bisphosphate drives Ca2+-independent membrane penetration by the tandem C2 domain proteins synaptotagmin-1 and Doc2β
- PMID: 31147445
- PMCID: PMC6635456
- DOI: 10.1074/jbc.RA119.007929
Phosphatidylinositol 4,5-bisphosphate drives Ca2+-independent membrane penetration by the tandem C2 domain proteins synaptotagmin-1 and Doc2β
Abstract
Exocytosis mediates the release of neurotransmitters and hormones from neurons and neuroendocrine cells. Tandem C2 domain proteins in the synaptotagmin (syt) and double C2 domain (Doc2) families regulate exocytotic membrane fusion via direct interactions with Ca2+ and phospholipid bilayers. Syt1 is a fast-acting, low-affinity Ca2+ sensor that penetrates membranes upon binding Ca2+ to trigger synchronous vesicle fusion. The closely related Doc2β is a slow-acting, high-affinity Ca2+ sensor that triggers spontaneous and asynchronous vesicle fusion, but whether it also penetrates membranes is unknown. Both syt1 and Doc2β bind the dynamically regulated plasma membrane lipid phosphatidylinositol 4,5-bisphosphate (PIP2), but it is unclear whether PIP2 serves only as a membrane contact or enables specialized membrane-binding modes by these Ca2+ sensors. Furthermore, it has been shown that PIP2 uncaging can trigger rapid, syt1-dependent exocytosis in the absence of Ca2+ influx, suggesting that current models for the action of these Ca2+ sensors are incomplete. Here, using a series of steady-state and time-resolved fluorescence measurements, we show that Doc2β, like syt1, penetrates membranes in a Ca2+-dependent manner. Unexpectedly, we observed that PIP2 can drive membrane penetration by both syt1 and Doc2β in the absence of Ca2+, providing a plausible mechanism for Ca2+-independent, PIP2-dependent exocytosis. Quantitative measurements of penetration depth revealed that, in the presence of Ca2+, PIP2 drives Doc2β, but not syt1, substantially deeper into the membrane, defining a biophysical regulatory mechanism specific to this high-affinity Ca2+ sensor. Our results provide evidence of a novel role for PIP2 in regulating, and under some circumstances triggering, exocytosis.
Keywords: calcium sensor; calcium-binding protein; exocytosis; membrane biophysics; membrane protein; phosphatidylinositol (4,5)-bisphosphate (PIP2); poly-anionic phospholipid; synapse; synaptotagmin; tandem C2 domain protein.
© 2019 Bradberry et al.
Conflict of interest statement
The authors declare that they have no conflicts of interest with the contents of this article
Figures
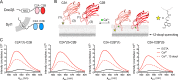
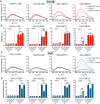
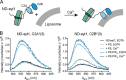
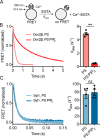
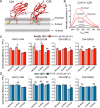
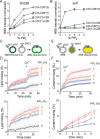
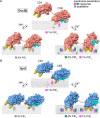
Similar articles
-
Allosteric stabilization of calcium and phosphoinositide dual binding engages several synaptotagmins in fast exocytosis.Elife. 2022 Aug 5;11:e74810. doi: 10.7554/eLife.74810. Elife. 2022. PMID: 35929728 Free PMC article.
-
Structural elements that underlie Doc2β function during asynchronous synaptic transmission.Proc Natl Acad Sci U S A. 2015 Aug 4;112(31):E4316-25. doi: 10.1073/pnas.1502288112. Epub 2015 Jul 20. Proc Natl Acad Sci U S A. 2015. PMID: 26195798 Free PMC article.
-
Mutations that disrupt Ca²⁺-binding activity endow Doc2β with novel functional properties during synaptic transmission.Mol Biol Cell. 2014 Feb;25(4):481-94. doi: 10.1091/mbc.E13-10-0571. Epub 2013 Dec 19. Mol Biol Cell. 2014. PMID: 24356452 Free PMC article.
-
Models of synaptotagmin-1 to trigger Ca2+ -dependent vesicle fusion.FEBS Lett. 2018 Nov;592(21):3480-3492. doi: 10.1002/1873-3468.13193. Epub 2018 Jul 30. FEBS Lett. 2018. PMID: 30004579 Review.
-
Exocytosis and synaptic vesicle function.Compr Physiol. 2014 Jan;4(1):149-75. doi: 10.1002/cphy.c130021. Compr Physiol. 2014. PMID: 24692137 Review.
Cited by
-
Cytotoxic Granule Trafficking and Fusion in Synaptotagmin7-Deficient Cytotoxic T Lymphocytes.Front Immunol. 2020 May 29;11:1080. doi: 10.3389/fimmu.2020.01080. eCollection 2020. Front Immunol. 2020. PMID: 32547563 Free PMC article.
-
Vesicle docking and fusion pore modulation by the neuronal calcium sensor Synaptotagmin-1.bioRxiv [Preprint]. 2024 Sep 12:2024.09.12.612660. doi: 10.1101/2024.09.12.612660. bioRxiv. 2024. Update in: Biophys J. 2024 Dec 23:S0006-3495(24)04104-3. doi: 10.1016/j.bpj.2024.12.023 PMID: 39314345 Free PMC article. Updated. Preprint.
-
Synaptotagmin-1 membrane binding is driven by the C2B domain and assisted cooperatively by the C2A domain.Sci Rep. 2020 Oct 22;10(1):18011. doi: 10.1038/s41598-020-74923-y. Sci Rep. 2020. PMID: 33093513 Free PMC article.
-
Differential SNARE chaperoning by Munc13-1 and Munc18-1 dictates fusion pore fate at the release site.Nat Commun. 2024 May 16;15(1):4132. doi: 10.1038/s41467-024-46965-7. Nat Commun. 2024. PMID: 38755165 Free PMC article.
-
The neuronal calcium sensor Synaptotagmin-1 and SNARE proteins cooperate to dilate fusion pores.Elife. 2021 Jun 30;10:e68215. doi: 10.7554/eLife.68215. Elife. 2021. PMID: 34190041 Free PMC article.
References
Publication types
MeSH terms
Substances
Associated data
- Actions
- Actions
- Actions
- Actions
Grants and funding
LinkOut - more resources
Full Text Sources
Research Materials
Miscellaneous