Changes of Thermostability, Organic Solvent, and pH Stability in Geobacillus zalihae HT1 and Its Mutant by Calcium Ion
- PMID: 31137725
- PMCID: PMC6566366
- DOI: 10.3390/ijms20102561
Changes of Thermostability, Organic Solvent, and pH Stability in Geobacillus zalihae HT1 and Its Mutant by Calcium Ion
Abstract
Thermostable T1 lipase from Geobacillus zalihae has been crystallized using counter-diffusion method under space and Earth conditions. The comparison of the three-dimensional structures from both crystallized proteins show differences in the formation of hydrogen bond and ion interactions. Hydrogen bond and ion interaction are important in the stabilization of protein structure towards extreme temperature and organic solvents. In this study, the differences of hydrogen bond interactions at position Asp43, Thr118, Glu250, and Asn304 and ion interaction at position Glu226 was chosen to imitate space-grown crystal structure, and the impact of these combined interactions in T1 lipase-mutated structure was studied. Using space-grown T1 lipase structure as a reference, subsequent simultaneous mutation D43E, T118N, E226D, E250L, and N304E was performed on recombinant wild-type T1 lipase (wt-HT1) to generate a quintuple mutant term as 5M mutant lipase. This mutant lipase shared similar characteristics to its wild-type in terms of optimal pH and temperature. The stability of mutant 5M lipase improved significantly in acidic and alkaline pH as compared to wt-HT1. 5M lipase was highly stable in organic solvents such as dimethyl sulfoxide (DMSO), methanol, and n-hexane compared to wt-HT1. Both wild-type and mutant lipases were found highly activated in calcium as compared to other metal ions due to the presence of calcium-binding site for thermostability. The presence of calcium prolonged the half-life of mutant 5M and wt-HT1, and at the same time increased their melting temperature (Tm). The melting temperature of 5M and wt-HT1 lipases increased at 8.4 and 12.1 °C, respectively, in the presence of calcium as compared to those without. Calcium enhanced the stability of mutant 5M in 25% (v/v) DMSO, n-hexane, and n-heptane. The lipase activity of wt-HT1 also increased in 25% (v/v) ethanol, methanol, acetonitrile, n-hexane, and n-heptane in the presence of calcium. The current study showed that the accumulation of amino acid substitutions D43E, T118N, E226D, E250L, and N304E produced highly stable T1 mutant when hydrolyzing oil in selected organic solvents such as DMSO, n-hexane, and n-heptane. It is also believed that calcium ion plays important role in regulating lipase thermostability.
Keywords: Calcium ion; Geobacillus zalihae; T1 lipase; metal-binding site; organic solvent tolerance; thermostability.
Conflict of interest statement
The authors declare no conflict of interest.
Figures
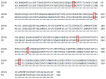
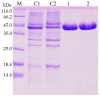
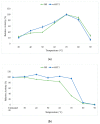
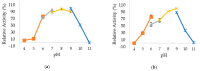
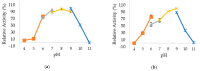
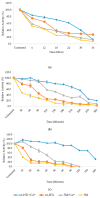
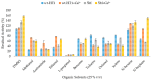
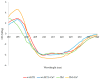
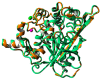
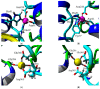
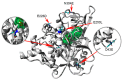
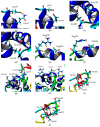
Similar articles
-
Structure elucidation and docking analysis of 5M mutant of T1 lipase Geobacillus zalihae.PLoS One. 2021 Jun 1;16(6):e0251751. doi: 10.1371/journal.pone.0251751. eCollection 2021. PLoS One. 2021. PMID: 34061877 Free PMC article.
-
Ion-Pair Interaction and Hydrogen Bonds as Main Features of Protein Thermostability in Mutated T1 Recombinant Lipase Originating from Geobacillus zalihae.Molecules. 2020 Jul 28;25(15):3430. doi: 10.3390/molecules25153430. Molecules. 2020. PMID: 32731607 Free PMC article.
-
Improvement of thermal stability via outer-loop ion pair interaction of mutated T1 lipase from Geobacillus zalihae strain T1.Int J Mol Sci. 2012;13(1):943-960. doi: 10.3390/ijms13010943. Epub 2012 Jan 17. Int J Mol Sci. 2012. PMID: 22312296 Free PMC article.
-
Engineering lipases for temperature adaptation: Structure function correlation.Biochim Biophys Acta Proteins Proteom. 2019 Nov;1867(11):140261. doi: 10.1016/j.bbapap.2019.08.001. Epub 2019 Aug 8. Biochim Biophys Acta Proteins Proteom. 2019. PMID: 31401312 Review.
-
Computer-Aided Lipase Engineering for Improving Their Stability and Activity in the Food Industry: State of the Art.Molecules. 2023 Aug 3;28(15):5848. doi: 10.3390/molecules28155848. Molecules. 2023. PMID: 37570817 Free PMC article. Review.
Cited by
-
Use of genomics & proteomics in studying lipase producing microorganisms & its application.Food Chem (Oxf). 2024 Aug 23;9:100218. doi: 10.1016/j.fochms.2024.100218. eCollection 2024 Dec 30. Food Chem (Oxf). 2024. PMID: 39281291 Free PMC article.
-
Enzymatic Performance of Aspergillus oryzae α-Amylase in the Presence of Organic Solvents: Activity, Stability, and Bioinformatic Studies.Bioinform Biol Insights. 2024 Apr 23;18:11779322241234767. doi: 10.1177/11779322241234767. eCollection 2024. Bioinform Biol Insights. 2024. PMID: 38660393 Free PMC article.
-
Structure elucidation and docking analysis of 5M mutant of T1 lipase Geobacillus zalihae.PLoS One. 2021 Jun 1;16(6):e0251751. doi: 10.1371/journal.pone.0251751. eCollection 2021. PLoS One. 2021. PMID: 34061877 Free PMC article.
-
A thermostable organic solvent-tolerant lipase from Brevibacillus sp.: production and integrated downstream processing using an alcohol-salt-based aqueous two-phase system.Front Microbiol. 2023 Oct 13;14:1270270. doi: 10.3389/fmicb.2023.1270270. eCollection 2023. Front Microbiol. 2023. PMID: 37901828 Free PMC article.
-
Ion-Pair Interaction and Hydrogen Bonds as Main Features of Protein Thermostability in Mutated T1 Recombinant Lipase Originating from Geobacillus zalihae.Molecules. 2020 Jul 28;25(15):3430. doi: 10.3390/molecules25153430. Molecules. 2020. PMID: 32731607 Free PMC article.
References
-
- Dong J., Pan J., Niu X., Zhou Y., Bi R. Influence of microgravity on protein crystal structures. Chin. Sci. Bull. 2000;45:1002–1006. doi: 10.1007/BF02884980. - DOI
MeSH terms
Substances
Grants and funding
LinkOut - more resources
Full Text Sources