Dual-Sized Microparticle System for Generating Suppressive Dendritic Cells Prevents and Reverses Type 1 Diabetes in the Nonobese Diabetic Mouse Model
- PMID: 31119191
- PMCID: PMC6518351
- DOI: 10.1021/acsbiomaterials.9b00332
Dual-Sized Microparticle System for Generating Suppressive Dendritic Cells Prevents and Reverses Type 1 Diabetes in the Nonobese Diabetic Mouse Model
Abstract
Antigen specificity is a primary goal in developing curative therapies for autoimmune disease. Dendritic cells (DCs), as the most effective antigen presenting cells in the body, represent a key target to mediate restoration of antigen-specific immune regulation. Here, we describe an injectable, dual-sized microparticle (MP) approach that employs phagocytosable ∼1 μm and nonphagocytosable ∼30 μm MPs to deliver tolerance-promoting factors both intracellularly and extracellularly, as well as the type 1 diabetes autoantigen, insulin, to DCs for reprogramming of immune responses and remediation of autoimmunity. This poly(lactic-co-glycolic acid) (PLGA) MP system prevented diabetes onset in 60% of nonobese diabetic (NOD) mice when administered subcutaneously in 8 week old mice. Prevention of disease was dependent upon antigen inclusion and required encapsulation of factors in MPs. Moreover, administration of this "suppressive-vaccine" boosted pancreatic lymph node and splenic regulatory T cells (Tregs), upregulated PD-1 on CD4+ and CD8+ T cells, and reversed hyperglycemia for up to 100 days in recent-onset NOD mice. Our results demonstrate that a MP-based platform can reeducate the immune system in an antigen-specific manner, augment immunomodulation compared to soluble administration of drugs, and provide a promising alternative to systemic immunosuppression for autoimmunity.
Conflict of interest statement
The authors declare the following competing financial interest(s): J.S.L. and G.P.M. conducted a portion of the reported experiments while employed by OneVax, LLC. C.H.W., T.M.B., M.A.A., and B.G.K. are cofounders of OneVax, LLC, a preclinical biotechnology company with interest in this technology. The authors declare that no other relevant conflicts of interest exist.
Figures
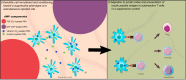
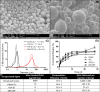
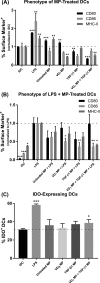
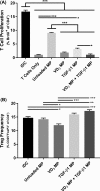
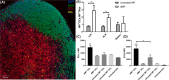
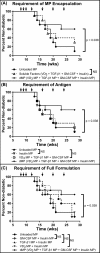
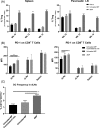
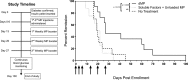
Similar articles
-
Combination Treatment with Antigen-Specific Dual-Sized Microparticle System Plus Anti-CD3 Immunotherapy Fails to Synergize to Improve Late-Stage Type 1 Diabetes Prevention in Nonobese Diabetic Mice.ACS Biomater Sci Eng. 2020 Oct 12;6(10):5941-5958. doi: 10.1021/acsbiomaterials.0c01075. Epub 2020 Sep 10. ACS Biomater Sci Eng. 2020. PMID: 33320581 Free PMC article.
-
A combination dual-sized microparticle system modulates dendritic cells and prevents type 1 diabetes in prediabetic NOD mice.Clin Immunol. 2015 Sep;160(1):90-102. doi: 10.1016/j.clim.2015.03.023. Epub 2015 Apr 2. Clin Immunol. 2015. PMID: 25842187 Free PMC article.
-
Combinatorial, Microparticle-Based Delivery of Immune Modulators Reprograms the Dendritic Cell Phenotype and Promotes Remission of Collagen-Induced Arthritis in Mice.ACS Appl Bio Mater. 2019 Jun 17;2(6):2388-2404. doi: 10.1021/acsabm.9b00092. Epub 2019 May 22. ACS Appl Bio Mater. 2019. PMID: 35030696
-
The interactions of dendritic cells with antigen-specific, regulatory T cells that suppress autoimmunity.Semin Immunol. 2006 Apr;18(2):93-102. doi: 10.1016/j.smim.2006.01.009. Epub 2006 Feb 15. Semin Immunol. 2006. PMID: 16469503 Review.
-
Control of type 1 autoimmune diabetes by naturally occurring CD4+CD25+ regulatory T lymphocytes in neonatal NOD mice.Ann N Y Acad Sci. 2005 Jun;1051:72-87. doi: 10.1196/annals.1361.048. Ann N Y Acad Sci. 2005. PMID: 16126946 Review.
Cited by
-
Antigen-specific T cell responses in autoimmune diabetes.Front Immunol. 2024 Aug 15;15:1440045. doi: 10.3389/fimmu.2024.1440045. eCollection 2024. Front Immunol. 2024. PMID: 39211046 Free PMC article. Review.
-
Biomaterial Strategies for Selective Immune Tolerance: Advances and Gaps.Adv Sci (Weinh). 2023 Mar;10(8):e2205105. doi: 10.1002/advs.202205105. Epub 2023 Jan 13. Adv Sci (Weinh). 2023. PMID: 36638260 Free PMC article. Review.
-
Inhibition of glycolysis in the presence of antigen generates suppressive antigen-specific responses and restrains rheumatoid arthritis in mice.Biomaterials. 2021 Oct;277:121079. doi: 10.1016/j.biomaterials.2021.121079. Epub 2021 Aug 20. Biomaterials. 2021. PMID: 34454372 Free PMC article.
-
Tissue-Targeted Drug Delivery Strategies to Promote Antigen-Specific Immune Tolerance.Adv Healthc Mater. 2023 Jan;12(6):e2202238. doi: 10.1002/adhm.202202238. Epub 2022 Dec 15. Adv Healthc Mater. 2023. PMID: 36417578 Free PMC article. Review.
-
Nanoparticles for Inducing Antigen-Specific T Cell Tolerance in Autoimmune Diseases.Front Immunol. 2022 Mar 22;13:864403. doi: 10.3389/fimmu.2022.864403. eCollection 2022. Front Immunol. 2022. PMID: 35392079 Free PMC article. Review.
References
-
- National Diabetes Statistics Report, 2014: Estimates of Diabetes and Its Burden in the United States; Centers for Disease Control and Prevention, U.S. Department of Health and Human Services: Atlanta, GA, 2014.
-
- Groop P. H.; Thomas M. C.; Moran J. L.; Waden J.; Thorn L. M.; Makinen V. P.; Rosengard-Barlund M.; Saraheimo M.; Hietala K.; Heikkila O.; Forsblom C. The presence and severity of chronic kidney disease predicts all-cause mortality in type 1 diabetes. Diabetes 2009, 58 (7), 1651–8. 10.2337/db08-1543. - DOI - PMC - PubMed
Grants and funding
LinkOut - more resources
Full Text Sources
Research Materials