Quantum dots in biomedical applications
- PMID: 31082570
- PMCID: PMC6642839
- DOI: 10.1016/j.actbio.2019.05.022
Quantum dots in biomedical applications
Abstract
Semiconducting nanoparticles, more commonly known as quantum dots, possess unique size and shape dependent optoelectronic properties. In recent years, these unique properties have attracted much attention in the biomedical field to enable real-time tissue imaging (bioimaging), diagnostics, single molecule probes, and drug delivery, among many other areas. The optical properties of quantum dots can be tuned by size and composition, and their high brightness, resistance to photobleaching, multiplexing capacity, and high surface-to-volume ratio make them excellent candidates for intracellular tracking, diagnostics, in vivo imaging, and therapeutic delivery. We discuss recent advances and challenges in the molecular design of quantum dots are discussed, along with applications of quantum dots as drug delivery vehicles, theranostic agents, single molecule probes, and real-time in vivo deep tissue imaging agents. We present a detailed discussion of the biodistribution and toxicity of quantum dots, and highlight recent advances to improve long-term stability in biological buffers, increase quantum yield following bioconjugation, and improve clearance from the body. Last, we present an outlook on future challenges and strategies to further advance translation to clinical application. STATEMENT OF SIGNIFICANCE: Semiconducting nanoparticles, commonly known as quantum dots, possess unique size and shape dependent electrical and optical properties. In recent years, they have attracted much attention in biomedical imaging to enable diagnostics, single molecule probes, and real-time imaging of tumors. This review discusses recent advances and challenges in the design of quantum dots, and highlights how these strategies can further advance translation to clinical applications.
Keywords: Bioimaging; Drug delivery; Molecular probes; Nanotechnology; Theranostics.
Copyright © 2019 Acta Materialia Inc. Published by Elsevier Ltd. All rights reserved.
Figures
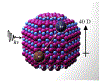
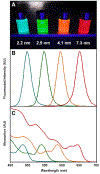
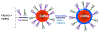
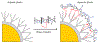
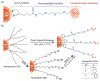
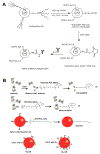
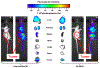
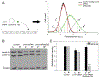
Similar articles
-
Aggregation-Induced Emission (AIE) Dots: Emerging Theranostic Nanolights.Acc Chem Res. 2018 Jun 19;51(6):1404-1414. doi: 10.1021/acs.accounts.8b00060. Epub 2018 May 7. Acc Chem Res. 2018. PMID: 29733571
-
Silicon Quantum Dots: Promising Theranostic Probes for the Future.Curr Drug Targets. 2019;20(12):1255-1263. doi: 10.2174/1389450120666190405152315. Curr Drug Targets. 2019. PMID: 30961492 Review.
-
Current Application of Quantum Dots (QD) in Cancer Therapy: A Review.Mini Rev Med Chem. 2017;17(14):1406-1415. doi: 10.2174/1389557517666170315125504. Mini Rev Med Chem. 2017. PMID: 28302038 Review.
-
Quantum dots in the biomedical world: A smart advanced nanocarrier for multiple venues application.Arch Pharm (Weinheim). 2022 Dec;355(12):e2200299. doi: 10.1002/ardp.202200299. Epub 2022 Sep 4. Arch Pharm (Weinheim). 2022. PMID: 36058643 Review.
-
Bioconjugated quantum dots for in vivo molecular and cellular imaging.Adv Drug Deliv Rev. 2008 Aug 17;60(11):1226-1240. doi: 10.1016/j.addr.2008.03.015. Epub 2008 Apr 10. Adv Drug Deliv Rev. 2008. PMID: 18495291 Free PMC article. Review.
Cited by
-
Highly Bright Silica-Coated InP/ZnS Quantum Dot-Embedded Silica Nanoparticles as Biocompatible Nanoprobes.Int J Mol Sci. 2022 Sep 19;23(18):10977. doi: 10.3390/ijms231810977. Int J Mol Sci. 2022. PMID: 36142888 Free PMC article.
-
Impact of Surface Trap States on Electron and Energy Transfer in CdSe Quantum Dots Studied by Femtosecond Transient Absorption Spectroscopy.Nanomaterials (Basel). 2023 Dec 22;14(1):34. doi: 10.3390/nano14010034. Nanomaterials (Basel). 2023. PMID: 38202489 Free PMC article.
-
Fabrication of biocompatible magneto-fluorescence nanoparticles as a platform for fluorescent sensor and magnetic hyperthermia applications.RSC Adv. 2021 Nov 1;11(56):35258-35267. doi: 10.1039/d1ra07389c. eCollection 2021 Oct 28. RSC Adv. 2021. PMID: 35493192 Free PMC article.
-
Synthesis, Properties and Bioimaging Applications of Silver-Based Quantum Dots.Int J Mol Sci. 2021 Nov 11;22(22):12202. doi: 10.3390/ijms222212202. Int J Mol Sci. 2021. PMID: 34830084 Free PMC article. Review.
-
Antifungal Nano-Therapy in Veterinary Medicine: Current Status and Future Prospects.J Fungi (Basel). 2021 Jun 22;7(7):494. doi: 10.3390/jof7070494. J Fungi (Basel). 2021. PMID: 34206304 Free PMC article. Review.
References
-
- Lim CT, Han J, Guck J, Espinosa H, Micro and nanotechnology for biological and biomedical applications, Med Biol Eng Comput 48(10) (2010) 941–943. - PubMed
-
- West JL, Halas NJ, ENGINEEREDNANOMATERIALS FORBIOPHOTONICSAPPLICATIONS: Improving Sensing, Imaging, and Therapeutics, Annu. Rev. Biomed. Eng 5(1) (2003) 285–292. - PubMed
Publication types
MeSH terms
Substances
Grants and funding
LinkOut - more resources
Full Text Sources
Research Materials