Passive force enhancement in striated muscle
- PMID: 31070958
- PMCID: PMC6620658
- DOI: 10.1152/japplphysiol.00676.2018
Passive force enhancement in striated muscle
Abstract
Passive force enhancement is defined as the increase in passive, steady-state, isometric force of an actively stretched muscle compared with the same muscle stretched passively to that same length. Passive force enhancement is long lasting, increases with increasing muscle length and increasing stretch magnitudes, contributes to the residual force enhancement in skeletal and cardiac muscle, and is typically only observed at muscle lengths at which passive forces occur naturally. Passive force enhancement is typically equal to or smaller than the total residual force enhancement, it persists when a muscle is deactivated and reactivated, but can be abolished instantaneously when a muscle is shortened quickly from its stretched length. There is strong evidence that the passive force enhancement is caused by the filamentous sarcomeric protein titin, although the detailed molecular mechanisms underlying passive force enhancement remain unknown. Here I propose a tentative mechanism based on experimental evidence that associates passive force enhancement with the shortening of titin's free spring length in the I-band region of sarcomeres. I suggest that this shortening is accomplished by titin binding to actin and that the trigger for titin-actin interactions is associated with the formation of strongly bound cross bridges between actin and myosin that exposes actin attachment sites for titin through movement of the regulatory proteins troponin and tropomyosin.
Keywords: cross bridge theory; residual force enhancement; sarcomere nonuniformity; sliding filament theory; titin.
Conflict of interest statement
No conflicts of interest, financial or otherwise, are declared by the author.
Figures
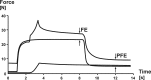
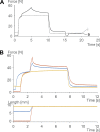
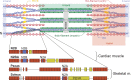
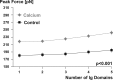
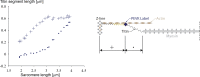
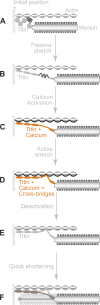
Similar articles
-
Differences in titin segmental elongation between passive and active stretch in skeletal muscle.J Exp Biol. 2017 Dec 1;220(Pt 23):4418-4425. doi: 10.1242/jeb.160762. Epub 2017 Oct 2. J Exp Biol. 2017. PMID: 28970245
-
Nonuniform elasticity of titin in cardiac myocytes: a study using immunoelectron microscopy and cellular mechanics.Biophys J. 1996 Jan;70(1):430-42. doi: 10.1016/S0006-3495(96)79586-3. Biophys J. 1996. PMID: 8770219 Free PMC article.
-
Force enhancement following stretch in a single sarcomere.Am J Physiol Cell Physiol. 2010 Dec;299(6):C1398-401. doi: 10.1152/ajpcell.00222.2010. Epub 2010 Sep 15. Am J Physiol Cell Physiol. 2010. PMID: 20844251
-
Mechanisms of enhanced force production in lengthening (eccentric) muscle contractions.J Appl Physiol (1985). 2014 Jun 1;116(11):1407-17. doi: 10.1152/japplphysiol.00069.2013. Epub 2013 Feb 21. J Appl Physiol (1985). 2014. PMID: 23429875 Review.
-
Sarcomere mechanics in striated muscles: from molecules to sarcomeres to cells.Am J Physiol Cell Physiol. 2017 Aug 1;313(2):C134-C145. doi: 10.1152/ajpcell.00050.2017. Epub 2017 May 24. Am J Physiol Cell Physiol. 2017. PMID: 28539306 Review.
Cited by
-
A three filament mechanistic model of musculotendon force and impedance.Elife. 2024 Sep 10;12:RP88344. doi: 10.7554/eLife.88344. Elife. 2024. PMID: 39254193 Free PMC article.
-
Linking in vivo muscle dynamics to force-length and force-velocity properties reveals that guinea fowl lateral gastrocnemius operates at shorter than optimal lengths.J Exp Biol. 2024 Aug 1;227(15):jeb246879. doi: 10.1242/jeb.246879. Epub 2024 Aug 1. J Exp Biol. 2024. PMID: 38873800
-
Male and female syringeal muscles exhibit superfast shortening velocities in zebra finches.J Exp Biol. 2024 Apr 1;227(7):jeb246330. doi: 10.1242/jeb.246330. Epub 2024 Apr 8. J Exp Biol. 2024. PMID: 38563308 Free PMC article.
-
A mechano- and heat-gated two-pore domain K+ channel controls excitability in adult zebrafish skeletal muscle.Proc Natl Acad Sci U S A. 2023 Nov 7;120(45):e2305959120. doi: 10.1073/pnas.2305959120. Epub 2023 Oct 30. Proc Natl Acad Sci U S A. 2023. PMID: 37903280 Free PMC article.
-
Skeletal muscle aging and sarcopenia: Perspectives from mechanical studies of single permeabilized muscle fibers.J Biomech. 2023 May;152:111559. doi: 10.1016/j.jbiomech.2023.111559. Epub 2023 Mar 27. J Biomech. 2023. PMID: 37027961 Free PMC article. Review.
References
-
- Avrova SV, Shelud’ko NS, Borovikov YS, Galler S. Twitchin of mollusc smooth muscles can induce “catch”-like properties in human skeletal muscle: support for the assumption that the “catch” state involves twitchin linkages between myofilaments. J Comp Physiol B 179: 945–950, 2009. doi:10.1007/s00360-009-0375-z. - DOI - PubMed
Publication types
MeSH terms
Substances
LinkOut - more resources
Full Text Sources