Pattern of cell-to-cell transfer of microRNA by gap junction and its effect on the proliferation of glioma cells
- PMID: 31012516
- PMCID: PMC6549926
- DOI: 10.1111/cas.14029
Pattern of cell-to-cell transfer of microRNA by gap junction and its effect on the proliferation of glioma cells
Abstract
MicroRNA is expected to be a novel therapeutic tool for tumors. Gap junctions facilitate the transfer of microRNA, which exerts biological effects on tumor cells. However, the length of microRNA that can pass through certain gap junctions composed of specific connexin remains unknown. To address this question, the present study investigated the permeability of gap junctions composed of various connexins, including connexin 43, connexin 32 or connexin 37, to microRNAs consisting of 18-27 nucleotides in glioma cells and cervical cancer cells. Results indicated that all of the microRNAs were able to be transferred from donor glioma cells to neighboring cells through the connexin 43 composed gap junction, but not the gap junctions composed of connexin 32 or connexin 37, in cervical cancer cells. Downregulation of the function of gap junctions comprising connexin 43 by pharmacological inhibition and shRNA significantly decreased the transfer of these microRNAs. In contrast, gap junction enhancers and overexpression of connexin 43 effectively increased these transfers. In glioma cells, cell proliferation was inhibited by microRNA-34a. Additionally, these effects of microRNA-34a were significantly enhanced by overexpression of connexin 43 in U251 cells, indicating that gap junctions play an important role in the antitumor effect of microRNA by transfer of microRNA to neighboring cells. Our data are the first to clarify the pattern of microRNA transmission through gap junctions and provide novel insights to show that antitumor microRNAs should be combined with connexin 43 or a connexin 43 enhancer, not connexin 32 or connexin 37, in order to improve the therapeutic effect.
Keywords: connexin; gap junction; glioma; microRNA; proliferation.
© 2019 The Authors. Cancer Science published by John Wiley & Sons Australia, Ltd on behalf of Japanese Cancer Association.
Figures
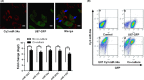
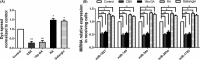
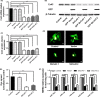
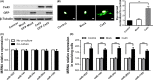
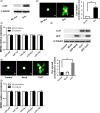
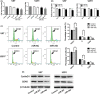
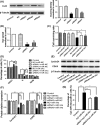
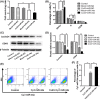
Similar articles
-
Gap junctions modulate glioma invasion by direct transfer of microRNA.Oncotarget. 2015 Jun 20;6(17):15566-77. doi: 10.18632/oncotarget.3904. Oncotarget. 2015. PMID: 25978028 Free PMC article.
-
Connexin-specific cell-to-cell transfer of short interfering RNA by gap junctions.J Physiol. 2005 Oct 15;568(Pt 2):459-68. doi: 10.1113/jphysiol.2005.090985. Epub 2005 Jul 21. J Physiol. 2005. PMID: 16037090 Free PMC article.
-
Vascular smooth muscle cell phenotypic transition regulates gap junctions of cardiomyocyte.Heart Vessels. 2020 Jul;35(7):1025-1035. doi: 10.1007/s00380-020-01602-3. Epub 2020 Apr 8. Heart Vessels. 2020. PMID: 32270355 Free PMC article.
-
Gap junction connexins in female reproductive organs: implications for women's reproductive health.Hum Reprod Update. 2015 May-Jun;21(3):340-52. doi: 10.1093/humupd/dmv007. Epub 2015 Feb 9. Hum Reprod Update. 2015. PMID: 25667189 Review.
-
Connexin 43 (Cx43) in cancer: Implications for therapeutic approaches via gap junctions.Cancer Lett. 2019 Feb 1;442:439-444. doi: 10.1016/j.canlet.2018.10.043. Epub 2018 Nov 22. Cancer Lett. 2019. PMID: 30472182 Review.
Cited by
-
Pathophysiology and pathogenic mechanisms of pulmonary hypertension: role of membrane receptors, ion channels, and Ca2+ signaling.Physiol Rev. 2023 Jul 1;103(3):1827-1897. doi: 10.1152/physrev.00030.2021. Epub 2022 Nov 24. Physiol Rev. 2023. PMID: 36422993 Free PMC article. Review.
-
MicroRNAs mediated interaction of tumor microenvironment cells with breast cancer cells during bone metastasis.Breast Cancer. 2023 Nov;30(6):910-925. doi: 10.1007/s12282-023-01491-0. Epub 2023 Aug 14. Breast Cancer. 2023. PMID: 37578597 Review.
-
Hypoxic Melanoma Cells Deliver microRNAs to Dendritic Cells and Cytotoxic T Lymphocytes through Connexin-43 Channels.Int J Mol Sci. 2020 Oct 13;21(20):7567. doi: 10.3390/ijms21207567. Int J Mol Sci. 2020. PMID: 33066331 Free PMC article.
-
The Roles of miRNA in Glioblastoma Tumor Cell Communication: Diplomatic and Aggressive Negotiations.Int J Mol Sci. 2020 Mar 12;21(6):1950. doi: 10.3390/ijms21061950. Int J Mol Sci. 2020. PMID: 32178454 Free PMC article. Review.
-
The intercellular communications mediating radiation-induced bystander effects and their relevance to environmental, occupational, and therapeutic exposures.Int J Radiat Biol. 2023;99(6):964-982. doi: 10.1080/09553002.2022.2078006. Epub 2022 May 27. Int J Radiat Biol. 2023. PMID: 35559659 Free PMC article. Review.
References
-
- Xu J, Zhao J, Evan G, Xiao C, Cheng Y, Xiao J. Circulating microRNAs: novel biomarkers for cardiovascular diseases. J Mol Med. 2012;90:865‐875. - PubMed
-
- Fu LL, Wen X, Bao JK, Liu B. MicroRNA‐modulated autophagic signaling networks in cancer. Int J Biochem Cell Biol. 2012;44:733‐736. - PubMed
-
- Hayes J, Peruzzi PP, Lawler S. MicroRNAs in cancer: biomarkers, functions and therapy. Trends Mol Med. 2014;20:460‐469. - PubMed
-
- Cheng G. Circulating miRNAs: roles in cancer diagnosis, prognosis and therapy. Adv Drug Deliv Rev. 2015;81:75‐93. - PubMed
MeSH terms
Substances
Grants and funding
- 16ykjc01/the Fundamental Research Funds for the Central Universities
- 81473234/National Natural Science Foundation of China
- 81373439/National Natural Science Foundation of China
- U1303221/the Joint Fund of the National Natural Science Foundation of China
- 20160908/Department of Science and Technology of Guangdong Province
LinkOut - more resources
Full Text Sources
Miscellaneous