TAF5L and TAF6L Maintain Self-Renewal of Embryonic Stem Cells via the MYC Regulatory Network
- PMID: 31005419
- PMCID: PMC6671628
- DOI: 10.1016/j.molcel.2019.03.025
TAF5L and TAF6L Maintain Self-Renewal of Embryonic Stem Cells via the MYC Regulatory Network
Abstract
Self-renewal and pluripotency of the embryonic stem cell (ESC) state are established and maintained by multiple regulatory networks that comprise transcription factors and epigenetic regulators. While much has been learned regarding transcription factors, the function of epigenetic regulators in these networks is less well defined. We conducted a CRISPR-Cas9-mediated loss-of-function genetic screen that identified two epigenetic regulators, TAF5L and TAF6L, components or co-activators of the GNAT-HAT complexes for the mouse ESC (mESC) state. Detailed molecular studies demonstrate that TAF5L/TAF6L transcriptionally activate c-Myc and Oct4 and their corresponding MYC and CORE regulatory networks. Besides, TAF5L/TAF6L predominantly regulate their target genes through H3K9ac deposition and c-MYC recruitment that eventually activate the MYC regulatory network for self-renewal of mESCs. Thus, our findings uncover a role of TAF5L/TAF6L in directing the MYC regulatory network that orchestrates gene expression programs to control self-renewal for the maintenance of mESC state.
Keywords: CRISPR; ESC; MYC; OCT4; TAF5L; TAF6L.
Copyright © 2019 Elsevier Inc. All rights reserved.
Conflict of interest statement
The authors declare no conflicts of interest.
Figures
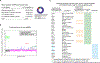
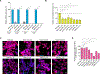
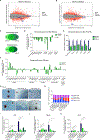
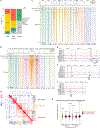
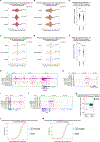
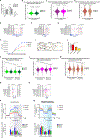
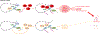
Similar articles
-
Polycomb Group Protein Pcgf6 Acts as a Master Regulator to Maintain Embryonic Stem Cell Identity.Sci Rep. 2016 Jun 1;6:26899. doi: 10.1038/srep26899. Sci Rep. 2016. PMID: 27247273 Free PMC article.
-
myc maintains embryonic stem cell pluripotency and self-renewal.Differentiation. 2010 Jul;80(1):9-19. doi: 10.1016/j.diff.2010.05.001. Epub 2010 May 27. Differentiation. 2010. PMID: 20537458 Free PMC article.
-
Stat3 and c-Myc genome-wide promoter occupancy in embryonic stem cells.PLoS One. 2008;3(12):e3932. doi: 10.1371/journal.pone.0003932. Epub 2008 Dec 11. PLoS One. 2008. PMID: 19079543 Free PMC article.
-
Transcriptional Control of Somatic Cell Reprogramming.Trends Cell Biol. 2016 Apr;26(4):272-288. doi: 10.1016/j.tcb.2015.12.003. Epub 2016 Jan 15. Trends Cell Biol. 2016. PMID: 26776886 Review.
-
A regulatory circuitry locking pluripotent stemness to embryonic stem cell: Interaction between threonine catabolism and histone methylation.Semin Cancer Biol. 2019 Aug;57:72-78. doi: 10.1016/j.semcancer.2019.01.005. Epub 2019 Jan 30. Semin Cancer Biol. 2019. PMID: 30710616 Review.
Cited by
-
Loss of Kat2a enhances transcriptional noise and depletes acute myeloid leukemia stem-like cells.Elife. 2020 Jan 27;9:e51754. doi: 10.7554/eLife.51754. Elife. 2020. PMID: 31985402 Free PMC article.
-
Histone H2Bub1 deubiquitylation is essential for mouse development, but does not regulate global RNA polymerase II transcription.Cell Death Differ. 2021 Aug;28(8):2385-2403. doi: 10.1038/s41418-021-00759-2. Epub 2021 Mar 17. Cell Death Differ. 2021. PMID: 33731875 Free PMC article.
-
Botanical Drug Puerarin Ameliorates Liposaccharide-Induced Depressive Behaviors in Mice via Inhibiting RagA/mTOR/p70S6K Pathways.Oxid Med Cell Longev. 2021 Oct 18;2021:7716201. doi: 10.1155/2021/7716201. eCollection 2021. Oxid Med Cell Longev. 2021. PMID: 34707778 Free PMC article.
-
Consistent DNA Hypomethylations in Prostate Cancer.Int J Mol Sci. 2022 Dec 26;24(1):386. doi: 10.3390/ijms24010386. Int J Mol Sci. 2022. PMID: 36613831 Free PMC article.
-
Decoding pluripotency: Genetic screens to interrogate the acquisition, maintenance, and exit of pluripotency.Wiley Interdiscip Rev Syst Biol Med. 2020 Jan;12(1):e1464. doi: 10.1002/wsbm.1464. Epub 2019 Aug 13. Wiley Interdiscip Rev Syst Biol Med. 2020. PMID: 31407519 Free PMC article. Review.
References
-
- Boyer LA, Plath K, Zeitlinger J, Brambrink T, Medeiros LA, Lee TI, Levine SS, Wernig M, Tajonar A, Ray MK, et al. (2006). Polycomb complexes repress developmental regulators in murine embryonic stem cells. Nature 441, 349–353. - PubMed
-
- Canver MC, Lessard S, Pinello L, Wu Y, Ilboudo Y, Stern EN, Needleman AJ, Galactéros F, Brugnara C, Kutlar A, et al. (2017). Variant-aware saturating mutagenesis using multiple Cas9 nucleases identifies regulatory elements at trait-associated loci. Nature Publishing Group 49, 625–634. - PMC - PubMed
Publication types
MeSH terms
Substances
Grants and funding
LinkOut - more resources
Full Text Sources
Molecular Biology Databases
Research Materials
Miscellaneous