Sirtuins and Type 2 Diabetes: Role in Inflammation, Oxidative Stress, and Mitochondrial Function
- PMID: 30972029
- PMCID: PMC6445872
- DOI: 10.3389/fendo.2019.00187
Sirtuins and Type 2 Diabetes: Role in Inflammation, Oxidative Stress, and Mitochondrial Function
Abstract
The rising incidence of type 2 diabetes mellitus (T2DM) is a major public health concern, and novel therapeutic strategies to prevent T2DM are urgently needed worldwide. Aging is recognized as one of the risk factors for metabolic impairments, including insulin resistance and T2DM. Inflammation, oxidative stress, and mitochondrial dysfunction are closely related to both aging and metabolic disease. Calorie restriction (CR) can retard the aging process in organisms ranging from yeast to rodents and delay the onset of numerous age-related disorders, such as insulin resistance and diabetes. Therefore, metabolic CR mimetics may represent new therapeutic targets for insulin resistance and T2DM. Sirtuin 1 (SIRT1), the mammalian homolog of Sir2, was originally identified as a nicotinamide adenine dinucleotide (NAD+)-dependent histone deacetylase. The activation of SIRT1 is closely associated with longevity under CR, and it is recognized as a CR mimetic. Currently, seven sirtuins have been identified in mammals. Among these sirtuins, SIRT1 and SIRT2 are located in the nucleus and cytoplasm, SIRT3 exists predominantly in mitochondria, and SIRT6 is located in the nucleus. These sirtuins regulate metabolism through their regulation of inflammation, oxidative stress and mitochondrial function via multiple mechanisms, resulting in the improvement of insulin resistance and T2DM. In this review, we describe the current understanding of the biological functions of sirtuins, especially SIRT1, SIRT2, SIRT3, and SIRT6, focusing on oxidative stress, inflammation, and mitochondrial function, which are closely associated with aging.
Keywords: SIRT1; SIRT2; SIRT3; SIRT6; Type 2 diabetes.
Figures
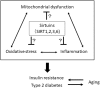
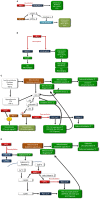
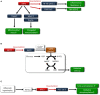
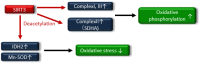
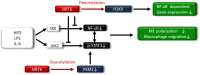
Similar articles
-
Sirtuins as possible drug targets in type 2 diabetes.Curr Drug Targets. 2013 Jun 1;14(6):622-36. doi: 10.2174/1389450111314060002. Curr Drug Targets. 2013. PMID: 23445543 Review.
-
The role of sirtuins in cardiac disease.Am J Physiol Heart Circ Physiol. 2015 Nov;309(9):H1375-89. doi: 10.1152/ajpheart.00053.2015. Epub 2015 Jul 31. Am J Physiol Heart Circ Physiol. 2015. PMID: 26232232 Free PMC article. Review.
-
SIRT1 in Type 2 Diabetes: Mechanisms and Therapeutic Potential.Diabetes Metab J. 2013 Oct;37(5):315-25. doi: 10.4093/dmj.2013.37.5.315. Diabetes Metab J. 2013. PMID: 24199159 Free PMC article. Review.
-
Sirtuins in gamete biology and reproductive physiology: emerging roles and therapeutic potential in female and male infertility.Hum Reprod Update. 2018 May 1;24(3):267-289. doi: 10.1093/humupd/dmy003. Hum Reprod Update. 2018. PMID: 29447380 Review.
-
Sirtuins and intervertebral disc degeneration: Roles in inflammation, oxidative stress, and mitochondrial function.Clin Chim Acta. 2020 Sep;508:33-42. doi: 10.1016/j.cca.2020.04.016. Epub 2020 Apr 26. Clin Chim Acta. 2020. PMID: 32348785 Review.
Cited by
-
Expression patterns of lncRNA MALAT-1 in SARS-COV-2 infection and its potential effect on disease severity via miR-200c-3p and SIRT1.Biochem Biophys Rep. 2023 Nov 1;36:101562. doi: 10.1016/j.bbrep.2023.101562. eCollection 2023 Dec. Biochem Biophys Rep. 2023. PMID: 37965063 Free PMC article.
-
Role of probiotics in managing various human diseases, from oral pathology to cancer and gastrointestinal diseases.Front Microbiol. 2024 Jan 5;14:1296447. doi: 10.3389/fmicb.2023.1296447. eCollection 2023. Front Microbiol. 2024. PMID: 38249451 Free PMC article. Review.
-
Mechanism of CD38 via NAD+ in the Development of Non-alcoholic Fatty Liver Disease.Int J Med Sci. 2023 Jan 22;20(2):262-266. doi: 10.7150/ijms.81381. eCollection 2023. Int J Med Sci. 2023. PMID: 36794157 Free PMC article. Review.
-
Metabolic reprogramming consequences of sepsis: adaptations and contradictions.Cell Mol Life Sci. 2022 Jul 29;79(8):456. doi: 10.1007/s00018-022-04490-0. Cell Mol Life Sci. 2022. PMID: 35904600 Free PMC article. Review.
-
Loss of SIRT1 in diabetes accelerates DNA damage-induced vascular calcification.Cardiovasc Res. 2021 Feb 22;117(3):836-849. doi: 10.1093/cvr/cvaa134. Cardiovasc Res. 2021. PMID: 32402066 Free PMC article.
References
Publication types
LinkOut - more resources
Full Text Sources