lncRedibly versatile: biochemical and biological functions of long noncoding RNAs
- PMID: 30971458
- PMCID: PMC6745715
- DOI: 10.1042/BCJ20180440
lncRedibly versatile: biochemical and biological functions of long noncoding RNAs
Abstract
Long noncoding RNAs (lncRNAs) are transcripts that do not code for proteins, but nevertheless exert regulatory effects on various biochemical pathways, in part via interactions with proteins, DNA, and other RNAs. LncRNAs are thought to regulate transcription and other biological processes by acting, for example, as guides that target proteins to chromatin, scaffolds that facilitate protein-protein interactions and complex formation, and orchestrators of phase-separated compartments. The study of lncRNAs has reached an exciting time, as recent advances in experimental and computational methods allow for genome-wide interrogation of biochemical and biological mechanisms of these enigmatic transcripts. A better appreciation for the biochemical versatility of lncRNAs has allowed us to begin closing gaps in our knowledge of how they act in diverse cellular and organismal contexts, including development and disease.
Keywords: RNA-binding proteins; chromatin; epigenetics; gene expression and regulation; large intervening noncoding RNA.
© 2019 The Author(s). Published by Portland Press Limited on behalf of the Biochemical Society.
Figures
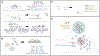
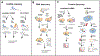
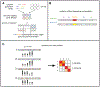
Similar articles
-
RNA-DNA Triplex Formation by Long Noncoding RNAs.Cell Chem Biol. 2016 Nov 17;23(11):1325-1333. doi: 10.1016/j.chembiol.2016.09.011. Epub 2016 Oct 20. Cell Chem Biol. 2016. PMID: 27773629 Review.
-
Perspectives on the mechanism of transcriptional regulation by long non-coding RNAs.Epigenetics. 2014 Jan;9(1):13-20. doi: 10.4161/epi.26700. Epub 2013 Oct 22. Epigenetics. 2014. PMID: 24149621 Free PMC article. Review.
-
RNA in unexpected places: long non-coding RNA functions in diverse cellular contexts.Nat Rev Mol Cell Biol. 2013 Nov;14(11):699-712. doi: 10.1038/nrm3679. Epub 2013 Oct 9. Nat Rev Mol Cell Biol. 2013. PMID: 24105322 Free PMC article. Review.
-
Long noncoding RNAs in lipid metabolism.Curr Opin Lipidol. 2018 Jun;29(3):224-232. doi: 10.1097/MOL.0000000000000503. Curr Opin Lipidol. 2018. PMID: 29553997 Free PMC article. Review.
-
Structure Prediction: New Insights into Decrypting Long Noncoding RNAs.Int J Mol Sci. 2016 Jan 21;17(1):132. doi: 10.3390/ijms17010132. Int J Mol Sci. 2016. PMID: 26805815 Free PMC article. Review.
Cited by
-
LncRNA GMDS-AS1 inhibits lung adenocarcinoma development by regulating miR-96-5p/CYLD signaling.Cancer Med. 2020 Feb;9(3):1196-1208. doi: 10.1002/cam4.2776. Epub 2019 Dec 20. Cancer Med. 2020. PMID: 31860169 Free PMC article.
-
Genome annotation with long RNA reads reveals new patterns of gene expression and improves single-cell analyses in an ant brain.BMC Biol. 2021 Nov 27;19(1):254. doi: 10.1186/s12915-021-01188-w. BMC Biol. 2021. PMID: 34838024 Free PMC article.
-
Long Non-Coding RNAs (lncRNAs) in Cardiovascular Disease Complication of Type 2 Diabetes.Diagnostics (Basel). 2021 Jan 19;11(1):145. doi: 10.3390/diagnostics11010145. Diagnostics (Basel). 2021. PMID: 33478141 Free PMC article. Review.
-
Circulating miRNAs and Preeclampsia: From Implantation to Epigenetics.Int J Mol Sci. 2024 Jan 24;25(3):1418. doi: 10.3390/ijms25031418. Int J Mol Sci. 2024. PMID: 38338700 Free PMC article. Review.
-
Synergistic combination of doxorubicin with hydralazine, and disulfiram against MCF-7 breast cancer cell line.PLoS One. 2023 Sep 28;18(9):e0291981. doi: 10.1371/journal.pone.0291981. eCollection 2023. PLoS One. 2023. PMID: 37768997 Free PMC article.
References
-
- Cech TR and Steitz JA (2014) The Noncoding RNA Revolution-Trashing Old Rules to Forge New Ones. Cell. 157, 77–94 - PubMed
-
- Kapranov P, Cawley SE, Drenkow J, Bekiranov S, Strausberg RL, Fodor SP and Gingeras TR (2002) Large-scale transcriptional activity in chromosomes 21 and 22. Science. 296, 916–919 - PubMed
-
- Okazaki Y, Furuno M, Kasukawa T, Adachi J, Bono H, Kondo S, Nikaido I, Osato N, Saito R, Suzuki H, Yamanaka I, Kiyosawa H, Yagi K, Tomaru Y, Hasegawa Y, Nogami A, Schonbach C, Gojobori T, Baldarelli R, Hill DP, Bult C, Hume DA, Quackenbush J, Schriml LM, Kanapin A, Matsuda H, Batalov S, Beisel KW, Blake JA, Bradt D, Brusic V, Chothia C, Corbani LE, Cousins S, Dalla E, Dragani TA, Fletcher CF, Forrest A, Frazer KS, Gaasterland T, Gariboldi M, Gissi C, Godzik A, Gough J, Grimmond S, Gustincich S, Hirokawa N, Jackson IJ, Jarvis ED, Kanai A, Kawaji H, Kawasawa Y, Kedzierski RM, King BL, Konagaya A, Kurochkin IV, Lee Y, Lenhard B, Lyons PA, Maglott DR, Maltais L, Marchionni L, McKenzie L, Miki H, Nagashima T, Numata K, Okido T, Pavan WJ, Pertea G, Pesole G, Petrovsky N, Pillai R, Pontius JU, Qi D, Ramachandran S, Ravasi T, Reed JC, Reed DJ, Reid J, Ring BZ, Ringwald M, Sandelin A, Schneider C, Semple CA, Setou M, Shimada K, Sultana R, Takenaka Y, Taylor MS, Teasdale RD, Tomita M, Verardo R, Wagner L, Wahlestedt C, Wang Y, Watanabe Y, Wells C, Wilming LG, Wynshaw-Boris A, Yanagisawa M, Yang I, Yang L, Yuan Z, Zavolan M, Zhu Y, Zimmer A, Carninci P, Hayatsu N, Hirozane-Kishikawa T, Konno H, Nakamura M, Sakazume N, Sato K, Shiraki T, Waki K, Kawai J, Aizawa K, Arakawa T, Fukuda S, Hara A, Hashizume W, Imotani K, Ishii Y, Itoh M, Kagawa I, Miyazaki A, Sakai K, Sasaki D, Shibata K, Shinagawa A, Yasunishi A, Yoshino M, Waterston R, Lander ES, Rogers J, Birney E, Hayashizaki Y, Consortium, F., I, R. G. E. R. G. P. and Team, I. I. (2002) Analysis of the mouse transcriptome based on functional annotation of 60,770 full-length cDNAs. Nature. 420, 563–573 - PubMed
-
- Bertone P, Stolc V, Royce TE, Rozowsky JS, Urban AE, Zhu X, Rinn JL, Tongprasit W, Samanta M, Weissman S, Gerstein M and Snyder M (2004) Global identification of human transcribed sequences with genome tiling arrays. Science. 306, 2242–2246 - PubMed
Publication types
MeSH terms
Substances
Grants and funding
LinkOut - more resources
Full Text Sources