Photo Processing for Biomedical Hydrogels Design and Functionality: A Review
- PMID: 30966045
- PMCID: PMC6415176
- DOI: 10.3390/polym10010011
Photo Processing for Biomedical Hydrogels Design and Functionality: A Review
Abstract
A large number of opportunities for biomedical hydrogel design and functionality through photo-processing have stretched the limits of innovation. As both photochemical understanding and engineering technologies continue to develop, more complicated geometries and spatiotemporal manipulations can be realized through photo-exposure, producing multifunctional hydrogels with specific chemical, biological and physical characteristics for the achievement of biomedical goals. This report describes the role that light has recently played in the synthesis and functionalization of biomedical hydrogels and primarily the design of photoresponsive hydrogels via different chemical reactions (photo crosslinking and photo degradation) and conventional light curing processes (micropatterning, stereolithography and two/multiphoton techniques) as well as typical biomedical applications of the hydrogels (cell culture, differentiation and in vivo vascularization) and their promising future.
Keywords: biomedical; hydrogels; photo crosslinking; photo degradation; photo dimerization.
Conflict of interest statement
The authors declare no conflicts of interest.
Figures
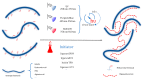
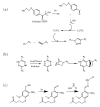
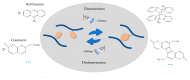
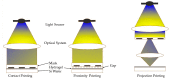
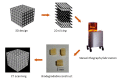
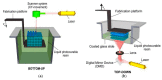
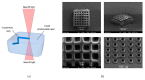
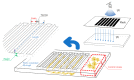
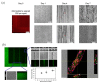
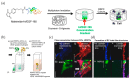
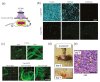
Similar articles
-
Recent advances in photo-crosslinkable hydrogels for biomedical applications.Biotechniques. 2019 Jan;66(1):40-53. doi: 10.2144/btn-2018-0083. Biotechniques. 2019. PMID: 30730212 Review.
-
Chemical gelling of hydrogels-based biological macromolecules for tissue engineering: Photo- and enzymatic-crosslinking methods.Int J Biol Macromol. 2019 Oct 15;139:760-772. doi: 10.1016/j.ijbiomac.2019.08.047. Epub 2019 Aug 7. Int J Biol Macromol. 2019. PMID: 31400425 Review.
-
Light manipulation for fabrication of hydrogels and their biological applications.Acta Biomater. 2022 Jan 1;137:20-43. doi: 10.1016/j.actbio.2021.10.003. Epub 2021 Oct 9. Acta Biomater. 2022. PMID: 34637933 Review.
-
Thermo-Responsive Hydrogels Coupled with Photothermal Agents for Biomedical Applications.Macromol Biosci. 2023 Dec;23(12):e2300214. doi: 10.1002/mabi.202300214. Epub 2023 Aug 17. Macromol Biosci. 2023. PMID: 37526220 Review.
-
In vitro evaluation of photo-crosslinkable chitosan-lactide hydrogels for bone tissue engineering.J Biomed Mater Res B Appl Biomater. 2014 Oct;102(7):1393-406. doi: 10.1002/jbm.b.33118. Epub 2014 Feb 6. J Biomed Mater Res B Appl Biomater. 2014. PMID: 24500890
Cited by
-
Photoresponsive hydrogel-based soft robot: A review.Mater Today Bio. 2023 May 10;20:100657. doi: 10.1016/j.mtbio.2023.100657. eCollection 2023 Jun. Mater Today Bio. 2023. PMID: 37229213 Free PMC article. Review.
-
Recent Progress and Trends in the Development of Electrospun and 3D Printed Polymeric-Based Materials to Overcome Antimicrobial Resistance (AMR).Pharmaceutics. 2023 Jul 16;15(7):1964. doi: 10.3390/pharmaceutics15071964. Pharmaceutics. 2023. PMID: 37514150 Free PMC article. Review.
-
Advancement in Soft Hydrogel Grippers: Comprehensive Insights into Materials, Fabrication Strategies, Grasping Mechanism, and Applications.Biomimetics (Basel). 2024 Sep 27;9(10):585. doi: 10.3390/biomimetics9100585. Biomimetics (Basel). 2024. PMID: 39451793 Free PMC article. Review.
-
Portable hand-held bioprinters promote in situ tissue regeneration.Bioeng Transl Med. 2022 Mar 10;7(3):e10307. doi: 10.1002/btm2.10307. eCollection 2022 Sep. Bioeng Transl Med. 2022. PMID: 36176625 Free PMC article. Review.
-
Characteristic and Chondrogenic Differentiation Analysis of Hybrid Hydrogels Comprised of Hyaluronic Acid Methacryloyl (HAMA), Gelatin Methacryloyl (GelMA), and the Acrylate-Functionalized Nano-Silica Crosslinker.Polymers (Basel). 2022 May 13;14(10):2003. doi: 10.3390/polym14102003. Polymers (Basel). 2022. PMID: 35631885 Free PMC article.
References
Publication types
LinkOut - more resources
Full Text Sources
Research Materials