Off the Clock: From Circadian Disruption to Metabolic Disease
- PMID: 30935034
- PMCID: PMC6480015
- DOI: 10.3390/ijms20071597
Off the Clock: From Circadian Disruption to Metabolic Disease
Abstract
Circadian timekeeping allows appropriate temporal regulation of an organism's internal metabolism to anticipate and respond to recurrent daily changes in the environment. Evidence from animal genetic models and from humans under circadian misalignment (such as shift work or jet lag) shows that disruption of circadian rhythms contributes to the development of obesity and metabolic disease. Inappropriate timing of food intake and high-fat feeding also lead to disruptions of the temporal coordination of metabolism and physiology and subsequently promote its pathogenesis. This review illustrates the impact of genetically or environmentally induced molecular clock disruption (at the level of the brain and peripheral tissues) and the interplay between the circadian system and metabolic processes. Here, we discuss some mechanisms responsible for diet-induced circadian desynchrony and consider the impact of nutritional cues in inter-organ communication, with a particular focus on the communication between peripheral organs and brain. Finally, we discuss the relay of environmental information by signal-dependent transcription factors to adjust the timing of gene oscillations. Collectively, a better knowledge of the mechanisms by which the circadian clock function can be compromised will lead to novel preventive and therapeutic strategies for obesity and other metabolic disorders arising from circadian desynchrony.
Keywords: adipose tissue; circadian rhythm; high-fat diet; metabolism; molecular clock; nutrients; obesity; suprachiasmatic nucleus.
Conflict of interest statement
The author declares no conflicts of interests.
Figures
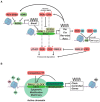
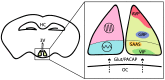
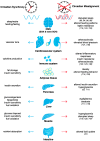
Similar articles
-
Metabolic implications of circadian disruption.Pflugers Arch. 2020 May;472(5):513-526. doi: 10.1007/s00424-020-02381-6. Epub 2020 May 4. Pflugers Arch. 2020. PMID: 32363530 Review.
-
Circadian timing of metabolism in animal models and humans.J Intern Med. 2015 May;277(5):513-27. doi: 10.1111/joim.12347. Epub 2015 Feb 6. J Intern Med. 2015. PMID: 25599827 Review.
-
Only time will tell: the interplay between circadian clock and metabolism.Chronobiol Int. 2021 Feb;38(2):149-167. doi: 10.1080/07420528.2020.1842436. Epub 2020 Dec 20. Chronobiol Int. 2021. PMID: 33345624 Free PMC article. Review.
-
Circadian Regulation of Metabolism: Commitment to Health and Diseases.Endocrinology. 2023 Jun 6;164(7):bqad086. doi: 10.1210/endocr/bqad086. Endocrinology. 2023. PMID: 37253106 Review.
-
Impact of nutrients on circadian rhythmicity.Am J Physiol Regul Integr Comp Physiol. 2015 Mar 1;308(5):R337-50. doi: 10.1152/ajpregu.00322.2014. Epub 2014 Dec 17. Am J Physiol Regul Integr Comp Physiol. 2015. PMID: 25519730 Free PMC article. Review.
Cited by
-
Beneficial Effects of Early Time-Restricted Feeding on Metabolic Diseases: Importance of Aligning Food Habits with the Circadian Clock.Nutrients. 2021 Apr 22;13(5):1405. doi: 10.3390/nu13051405. Nutrients. 2021. PMID: 33921979 Free PMC article. Review.
-
Circadian disruption, clock genes, and metabolic health.J Clin Invest. 2024 Jul 15;134(14):e170998. doi: 10.1172/JCI170998. J Clin Invest. 2024. PMID: 39007272 Free PMC article. Review.
-
Circadian Rhythm Disruption Influenced Hepatic Lipid Metabolism, Gut Microbiota and Promoted Cholesterol Gallstone Formation in Mice.Front Endocrinol (Lausanne). 2021 Oct 21;12:723918. doi: 10.3389/fendo.2021.723918. eCollection 2021. Front Endocrinol (Lausanne). 2021. PMID: 34745000 Free PMC article.
-
REV-ERB nuclear receptors in the suprachiasmatic nucleus control circadian period and restrict diet-induced obesity.Sci Adv. 2021 Oct 29;7(44):eabh2007. doi: 10.1126/sciadv.abh2007. Epub 2021 Oct 27. Sci Adv. 2021. PMID: 34705514 Free PMC article.
-
Living at the Wrong Time: Effects of Unmatching Official Time in Portugal and Western Spain.Biology (Basel). 2022 Jul 28;11(8):1130. doi: 10.3390/biology11081130. Biology (Basel). 2022. PMID: 36009758 Free PMC article.
References
Publication types
MeSH terms
Substances
Grants and funding
LinkOut - more resources
Full Text Sources
Medical