Biased Signaling of the Mu Opioid Receptor Revealed in Native Neurons
- PMID: 30925410
- PMCID: PMC6439305
- DOI: 10.1016/j.isci.2019.03.011
Biased Signaling of the Mu Opioid Receptor Revealed in Native Neurons
Abstract
G protein-coupled receptors are key signaling molecules and major targets for pharmaceuticals. The concept of ligand-dependent biased signaling raises the possibility of developing drugs with improved efficacy and safety profiles, yet translating this concept to native tissues remains a major challenge. Whether drug activity profiling in recombinant cell-based assays, traditionally used for drug discovery, has any relevance to physiology is unknown. Here we focused on the mu opioid receptor, the unrivalled target for pain treatment and also the key driver for the current opioid crisis. We selected a set of clinical and novel mu agonists, and profiled their activities in transfected cell assays using advanced biosensors and in native neurons from knock-in mice expressing traceable receptors endogenously. Our data identify Gi-biased agonists, including buprenorphine, and further show highly correlated drug activities in the two otherwise very distinct experimental systems, supporting in vivo translatability of biased signaling for mu opioid drugs.
Keywords: Bioengineering; Biological Sciences; Cell Biology; Molecular Biology; Neuroscience; Physiology.
Copyright © 2019 The Authors. Published by Elsevier Inc. All rights reserved.
Figures
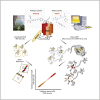
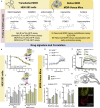
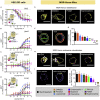
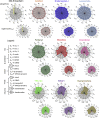
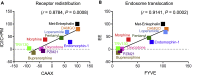
Similar articles
-
A Biased View of μ-Opioid Receptors?Mol Pharmacol. 2019 Nov;96(5):542-549. doi: 10.1124/mol.119.115956. Epub 2019 Jun 7. Mol Pharmacol. 2019. PMID: 31175184 Free PMC article. Review.
-
Fulfilling the Promise of "Biased" G Protein-Coupled Receptor Agonism.Mol Pharmacol. 2015 Sep;88(3):579-88. doi: 10.1124/mol.115.099630. Epub 2015 Jul 1. Mol Pharmacol. 2015. PMID: 26134495 Free PMC article. Review.
-
Structure-activity relationships and discovery of a G protein biased μ opioid receptor ligand, [(3-methoxythiophen-2-yl)methyl]({2-[(9R)-9-(pyridin-2-yl)-6-oxaspiro-[4.5]decan-9-yl]ethyl})amine (TRV130), for the treatment of acute severe pain.J Med Chem. 2013 Oct 24;56(20):8019-31. doi: 10.1021/jm4010829. Epub 2013 Oct 14. J Med Chem. 2013. PMID: 24063433
-
In vitro and in vivo activity of cyclopeptide Dmt-c[d-Lys-Phe-Asp]NH2, a mu opioid receptor agonist biased toward β-arrestin.Peptides. 2018 Jul;105:51-57. doi: 10.1016/j.peptides.2018.04.014. Epub 2018 Apr 22. Peptides. 2018. PMID: 29684591
-
Mu-Opioid receptor biased ligands: A safer and painless discovery of analgesics?Drug Discov Today. 2017 Nov;22(11):1719-1729. doi: 10.1016/j.drudis.2017.07.002. Epub 2017 Jul 22. Drug Discov Today. 2017. PMID: 28743488 Free PMC article. Review.
Cited by
-
Functional expression of opioid receptors and other human GPCRs in yeast engineered to produce human sterols.Nat Commun. 2022 May 24;13(1):2882. doi: 10.1038/s41467-022-30570-7. Nat Commun. 2022. PMID: 35610225 Free PMC article.
-
Molecular insights into the biased signaling mechanism of the μ-opioid receptor.Mol Cell. 2021 Oct 21;81(20):4165-4175.e6. doi: 10.1016/j.molcel.2021.07.033. Epub 2021 Aug 24. Mol Cell. 2021. PMID: 34433090 Free PMC article.
-
Exploring Pharmacological Functions of Alternatively Spliced Variants of the Mu Opioid Receptor Gene, Oprm1, via Gene-Targeted Animal Models.Int J Mol Sci. 2022 Mar 10;23(6):3010. doi: 10.3390/ijms23063010. Int J Mol Sci. 2022. PMID: 35328429 Free PMC article. Review.
-
Structures and Dynamics of Native-State Transmembrane Protein Targets and Bound Lipids.Membranes (Basel). 2021 Jun 17;11(6):451. doi: 10.3390/membranes11060451. Membranes (Basel). 2021. PMID: 34204456 Free PMC article. Review.
-
Knock-In Mouse Models to Investigate the Functions of Opioid Receptors in vivo.Front Cell Neurosci. 2022 Jan 31;16:807549. doi: 10.3389/fncel.2022.807549. eCollection 2022. Front Cell Neurosci. 2022. PMID: 35173584 Free PMC article. Review.
References
-
- Ayanga D., Shorter D., Kosten T.R. Update on pharmacotherapy for treatment of opioid use disorder. Expert Opin. Pharmacother. 2016;17:2307–2318. - PubMed
-
- Black J.W., Leff P. Operational models of pharmacological agonism. Proc. R. Soc. Lond. B Biol. Sci. 1983;220:141–162. - PubMed
-
- Breton B., Lagace M., Bouvier M. Combining resonance energy transfer methods reveals a complex between the alpha2A-adrenergic receptor, Galphai1beta1gamma2, and GRK2. FASEB J. 2010;24:4733–4743. - PubMed
-
- Compton W.M., Jones C.M., Baldwin G.T. Relationship between nonmedical prescription-opioid use and heroin use. N. Engl. J. Med. 2016;374:154–163. - PubMed
-
- DeWire S.M., Yamashita D.S., Rominger D.H., Liu G., Cowan C.L., Graczyk T.M., Chen X.T., Pitis P.M., Gotchev D., Yuan C. A G protein-biased ligand at the mu-opioid receptor is potently analgesic with reduced gastrointestinal and respiratory dysfunction compared with morphine. J. Pharmacol. Exp. Ther. 2013;344:708–717. - PubMed
Grants and funding
LinkOut - more resources
Full Text Sources
Other Literature Sources
Molecular Biology Databases
Research Materials