A Pathogen and a Non-pathogen Spotted Fever Group Rickettsia Trigger Differential Proteome Signatures in Macrophages
- PMID: 30895174
- PMCID: PMC6414445
- DOI: 10.3389/fcimb.2019.00043
A Pathogen and a Non-pathogen Spotted Fever Group Rickettsia Trigger Differential Proteome Signatures in Macrophages
Abstract
We have previously reported that Rickettsia conorii and Rickettsia montanensis have distinct intracellular fates within THP-1 macrophages, suggesting that the ability to proliferate within macrophages may be a distinguishable factor between pathogenic and non-pathogenic Spotted fever group (SFG) members. To start unraveling the molecular mechanisms underlying the capacity (or not) of SFG Rickettsia to establish their replicative niche in macrophages, we have herein used quantitative proteomics by SWATH-MS to profile the alterations resulted by the challenge of THP-1 macrophages with R. conorii and R. montanensis. We show that the pathogenic, R. conorii, and the non-pathogenic, R. montanensis, member of SFG Rickettsia trigger differential proteomic signatures in macrophage-like cells upon infection. R. conorii specifically induced the accumulation of several enzymes of the tricarboxylic acid cycle, oxidative phosphorylation, fatty acid β-oxidation, and glutaminolysis, as well as of several inner and outer membrane mitochondrial transporters. These results suggest a profound metabolic rewriting of macrophages by R. conorii toward a metabolic signature of an M2-like, anti-inflammatory activation program. Moreover, several subunits forming the proteasome and immunoproteasome are found in lower abundance upon infection with both rickettsial species, which may help bacteria to escape immune surveillance. R. conorii-infection specifically induced the accumulation of several host proteins implicated in protein processing and quality control in ER, suggesting that this pathogenic Rickettsia may be able to increase the ER protein folding capacity. This work reveals novel aspects of macrophage-Rickettsia interactions, expanding our knowledge of how pathogenic rickettsiae explore host cells to their advantage.
Keywords: Rickettsia conorii; Rickettsia montanensis; SWATH-MS; host-pathogen interactions; macrophages; metabolic reprogramming; protein processing pathways; spotted fever group Rickettsia.
Figures
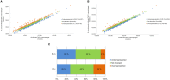
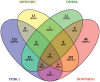
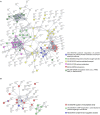
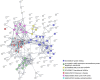
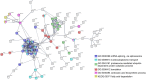
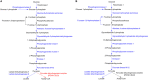
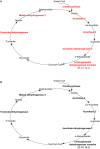

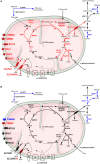
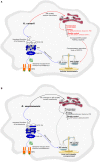
Similar articles
-
Macrophages Infected by a Pathogen and a Non-pathogen Spotted Fever Group Rickettsia Reveal Differential Reprogramming Signatures Early in Infection.Front Cell Infect Microbiol. 2019 Apr 10;9:97. doi: 10.3389/fcimb.2019.00097. eCollection 2019. Front Cell Infect Microbiol. 2019. PMID: 31024862 Free PMC article.
-
Differences in Intracellular Fate of Two Spotted Fever Group Rickettsia in Macrophage-Like Cells.Front Cell Infect Microbiol. 2016 Jul 29;6:80. doi: 10.3389/fcimb.2016.00080. eCollection 2016. Front Cell Infect Microbiol. 2016. PMID: 27525249 Free PMC article.
-
Spotted Fever Group Rickettsia Trigger Species-Specific Alterations in Macrophage Proteome Signatures with Different Impacts in Host Innate Inflammatory Responses.Microbiol Spectr. 2021 Dec 22;9(3):e0081421. doi: 10.1128/spectrum.00814-21. Epub 2021 Dec 22. Microbiol Spectr. 2021. PMID: 34935429 Free PMC article.
-
Historical and recent evidence for close relationships among Rickettsia parkeri, R. conorii, R. africae, and R. sibirica: implications for rickettsial taxonomy.J Vector Ecol. 2009 Dec;34(2):238-42. doi: 10.1111/j.1948-7134.2009.00032.x. J Vector Ecol. 2009. PMID: 20836828 Review.
-
[Emerging rickettsioses].Parassitologia. 2004 Jun;46(1-2):123-6. Parassitologia. 2004. PMID: 15305700 Review. Italian.
Cited by
-
An Indispensable Role for the MavE Effector of Legionella pneumophila in Lysosomal Evasion.mBio. 2021 Feb 9;12(1):e03458-20. doi: 10.1128/mBio.03458-20. mBio. 2021. PMID: 33563829 Free PMC article.
-
Idiosyncratic Biogenesis of Intracellular Pathogens-Containing Vacuoles.Front Cell Infect Microbiol. 2021 Nov 11;11:722433. doi: 10.3389/fcimb.2021.722433. eCollection 2021. Front Cell Infect Microbiol. 2021. PMID: 34858868 Free PMC article. Review.
-
Rickettsia conorii survival in THP-1 macrophages involves host lipid droplet alterations and active rickettsial protein production.Cell Microbiol. 2021 Nov;23(11):e13390. doi: 10.1111/cmi.13390. Epub 2021 Sep 13. Cell Microbiol. 2021. PMID: 34464019 Free PMC article.
-
Rickettsia-Host-Tick Interactions: Knowledge Advances and Gaps.Infect Immun. 2022 Sep 15;90(9):e0062121. doi: 10.1128/iai.00621-21. Epub 2022 Aug 22. Infect Immun. 2022. PMID: 35993770 Free PMC article. Review.
-
Host Epigenetics in Intracellular Pathogen Infections.Int J Mol Sci. 2020 Jun 27;21(13):4573. doi: 10.3390/ijms21134573. Int J Mol Sci. 2020. PMID: 32605029 Free PMC article. Review.
References
Publication types
MeSH terms
Substances
Grants and funding
LinkOut - more resources
Full Text Sources
Molecular Biology Databases