Altered Regulation of Striatal Neuronal N-Methyl-D-Aspartate Receptor Trafficking by Palmitoylation in Huntington Disease Mouse Model
- PMID: 30846936
- PMCID: PMC6393405
- DOI: 10.3389/fnsyn.2019.00003
Altered Regulation of Striatal Neuronal N-Methyl-D-Aspartate Receptor Trafficking by Palmitoylation in Huntington Disease Mouse Model
Abstract
N-methyl-D-aspartate receptors (NMDARs) play a critical role in synaptic signaling, and alterations in the synaptic/extrasynaptic NMDAR balance affect neuronal survival. Studies have shown enhanced extrasynaptic GluN2B-type NMDAR (2B-NMDAR) activity in striatal neurons in the YAC128 mouse model of Huntington disease (HD), resulting in increased cell death pathway activation contributing to striatal vulnerability to degeneration. However, the mechanism(s) of altered GluN2B trafficking remains unclear. Previous work shows that GluN2B palmitoylation on two C-terminal cysteine clusters regulates 2B-NMDAR trafficking to the surface membrane and synapses in cortical neurons. Notably, two palmitoyl acyltransferases (PATs), zDHHC17 and zDHHC13, also called huntingtin-interacting protein 14 (HIP14) and HIP14-like (HIP14L), directly interact with the huntingtin protein (Htt), and mutant Htt disrupts this interaction. Here, we investigated whether GluN2B palmitoylation is involved in enhanced extrasynaptic surface expression of 2B-NMDARs in YAC128 striatal neurons and whether this process is regulated by HIP14 or HIP14L. We found reduced GluN2B palmitoylation in YAC128 striatum, specifically on cysteine cluster II. Consistent with that finding, the palmitoylation-deficient GluN2B Cysteine cluster II mutant exhibited enhanced, extrasynaptic surface expression in striatal neurons from wild-type mice, mimicking increased extrasynaptic 2B-NMDAR observed in YAC128 cultures. We also found that HIP14L palmitoylated GluN2B cysteine cluster II. Moreover, GluN2B palmitoylation levels were reduced in striatal tissue from HIP14L-deficient mice, and siRNA-mediated HIP14L knockdown in cultured neurons enhanced striatal neuronal GluN2B surface expression and susceptibility to NMDA toxicity. Thus, altered regulation of GluN2B palmitoylation levels by the huntingtin-associated PAT HIP14L may contribute to the cell death-signaling pathways underlying HD.
Keywords: Huntington disease (HD); NMDAR palmitoylation; huntingtin interacting protein 14 (HIP14); huntingtin interacting protein 14-like (HIP14L); palmitoyl acyltransferase (PAT).
Figures
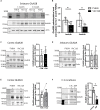
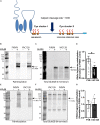
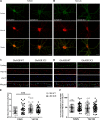
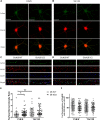
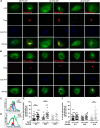
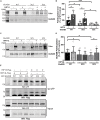
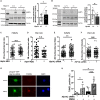
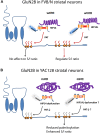








Similar articles
-
Hip14l-deficient mice develop neuropathological and behavioural features of Huntington disease.Hum Mol Genet. 2013 Feb 1;22(3):452-65. doi: 10.1093/hmg/dds441. Epub 2012 Oct 16. Hum Mol Genet. 2013. PMID: 23077216
-
Huntingtin interacting proteins 14 and 14-like are required for chorioallantoic fusion during early placental development.Dev Biol. 2015 Jan 15;397(2):257-66. doi: 10.1016/j.ydbio.2014.11.018. Epub 2014 Dec 3. Dev Biol. 2015. PMID: 25478910
-
Calpain and STriatal-Enriched protein tyrosine phosphatase (STEP) activation contribute to extrasynaptic NMDA receptor localization in a Huntington's disease mouse model.Hum Mol Genet. 2012 Sep 1;21(17):3739-52. doi: 10.1093/hmg/dds154. Epub 2012 Apr 20. Hum Mol Genet. 2012. PMID: 22523092 Free PMC article.
-
Aberrant palmitoylation in Huntington disease.Biochem Soc Trans. 2015 Apr;43(2):205-10. doi: 10.1042/BST20140242. Biochem Soc Trans. 2015. PMID: 25849918 Review.
-
Putting proteins in their place: palmitoylation in Huntington disease and other neuropsychiatric diseases.Prog Neurobiol. 2012 May;97(2):220-38. doi: 10.1016/j.pneurobio.2011.11.002. Epub 2011 Dec 7. Prog Neurobiol. 2012. PMID: 22155432 Review.
Cited by
-
Heavy Metal Interactions with Neuroglia and Gut Microbiota: Implications for Huntington's Disease.Cells. 2024 Jul 3;13(13):1144. doi: 10.3390/cells13131144. Cells. 2024. PMID: 38994995 Free PMC article. Review.
-
The role of s-palmitoylation in neurological diseases: implication for zDHHC family.Front Pharmacol. 2024 Jan 16;14:1342830. doi: 10.3389/fphar.2023.1342830. eCollection 2023. Front Pharmacol. 2024. PMID: 38293675 Free PMC article. Review.
-
Huntington's Disease: Complex Pathogenesis and Therapeutic Strategies.Int J Mol Sci. 2024 Mar 29;25(7):3845. doi: 10.3390/ijms25073845. Int J Mol Sci. 2024. PMID: 38612657 Free PMC article. Review.
-
Molecular Pathophysiological Mechanisms in Huntington's Disease.Biomedicines. 2022 Jun 17;10(6):1432. doi: 10.3390/biomedicines10061432. Biomedicines. 2022. PMID: 35740453 Free PMC article. Review.
-
Limb-Clasping Response in NMDA Receptor Palmitoylation-Deficient Mice.Mol Neurobiol. 2024 Nov;61(11):9125-9135. doi: 10.1007/s12035-024-04166-9. Epub 2024 Apr 9. Mol Neurobiol. 2024. PMID: 38592586 Free PMC article.
References
LinkOut - more resources
Full Text Sources
Molecular Biology Databases