Functional Morphology of the Cardiac Jelly in the Tubular Heart of Vertebrate Embryos
- PMID: 30818886
- PMCID: PMC6463132
- DOI: 10.3390/jcdd6010012
Functional Morphology of the Cardiac Jelly in the Tubular Heart of Vertebrate Embryos
Abstract
The early embryonic heart is a multi-layered tube consisting of (1) an outer myocardial tube; (2) an inner endocardial tube; and (3) an extracellular matrix layer interposed between the myocardium and endocardium, called "cardiac jelly" (CJ). During the past decades, research on CJ has mainly focused on its molecular and cellular biological aspects. This review focuses on the morphological and biomechanical aspects of CJ. Special attention is given to (1) the spatial distribution and fiber architecture of CJ; (2) the morphological dynamics of CJ during the cardiac cycle; and (3) the removal/remodeling of CJ during advanced heart looping stages, which leads to the formation of ventricular trabeculations and endocardial cushions. CJ acts as a hydraulic skeleton, displaying striking structural and functional similarities with the mesoglea of jellyfish. CJ not only represents a filler substance, facilitating end-systolic occlusion of the embryonic heart lumen. Its elastic components antagonize the systolic deformations of the heart wall and thereby power the refilling phase of the ventricular tube. Non-uniform spatial distribution of CJ generates non-circular cross sections of the opened endocardial tube (initially elliptic, later deltoid), which seem to be advantageous for valveless pumping. Endocardial cushions/ridges are cellularized remnants of non-removed CJ.
Keywords: ballooning; blood flow; cardiac jelly; embryonic heart tube; extracellular matrix; heart skeleton; hydraulic skeleton; non-circular cross sections; trabeculation; valveless pumping.
Conflict of interest statement
The authors declare no conflict of interest.
Figures
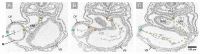
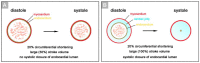
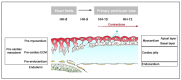
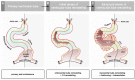
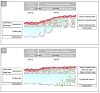
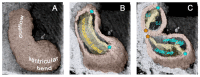
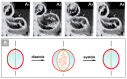
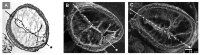
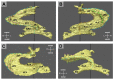
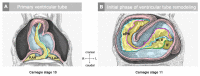
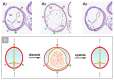
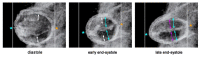
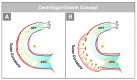
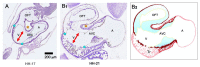
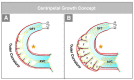
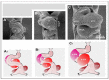
Similar articles
-
High-resolution in vivo imaging of the cross-sectional deformations of contracting embryonic heart loops using optical coherence tomography.Dev Dyn. 2008 Apr;237(4):953-61. doi: 10.1002/dvdy.21483. Dev Dyn. 2008. PMID: 18330931
-
In vivo imaging of the cyclic changes in cross-sectional shape of the ventricular segment of pulsating embryonic chick hearts at stages 14 to 17: a contribution to the understanding of the ontogenesis of cardiac pumping function.Dev Dyn. 2009 Dec;238(12):3273-84. doi: 10.1002/dvdy.22159. Dev Dyn. 2009. PMID: 19924823
-
Cardiac jelly arrangement during the formation of the tubular heart of the chick embryo.Acta Anat (Basel). 1977;98(4):444-5. doi: 10.1159/000144824. Acta Anat (Basel). 1977. PMID: 142414
-
How does the tubular embryonic heart work? Looking for the physical mechanism generating unidirectional blood flow in the valveless embryonic heart tube.Dev Dyn. 2010 Apr;239(4):1035-46. doi: 10.1002/dvdy.22265. Dev Dyn. 2010. PMID: 20235196 Review.
-
Cardiac looping in the chick embryo: a morphological review with special reference to terminological and biomechanical aspects of the looping process.Anat Rec. 2000 Jul 1;259(3):248-62. doi: 10.1002/1097-0185(20000701)259:3<248::AID-AR30>3.0.CO;2-K. Anat Rec. 2000. PMID: 10861359 Review.
Cited by
-
Following the Beat: Imaging the Valveless Pumping Function in the Early Embryonic Heart.J Cardiovasc Dev Dis. 2022 Aug 15;9(8):267. doi: 10.3390/jcdd9080267. J Cardiovasc Dev Dis. 2022. PMID: 36005431 Free PMC article. Review.
-
Zebrafish as a Model to Study Vascular Elastic Fibers and Associated Pathologies.Int J Mol Sci. 2022 Feb 14;23(4):2102. doi: 10.3390/ijms23042102. Int J Mol Sci. 2022. PMID: 35216218 Free PMC article. Review.
-
Reptiles as a Model System to Study Heart Development.Cold Spring Harb Perspect Biol. 2020 May 1;12(5):a037226. doi: 10.1101/cshperspect.a037226. Cold Spring Harb Perspect Biol. 2020. PMID: 31712265 Free PMC article. Review.
-
Dual role for CXCL12 signaling in semilunar valve development.Cell Rep. 2021 Aug 24;36(8):109610. doi: 10.1016/j.celrep.2021.109610. Cell Rep. 2021. PMID: 34433040 Free PMC article.
-
Bearing My Heart: The Role of Extracellular Matrix on Cardiac Development, Homeostasis, and Injury Response.Front Cell Dev Biol. 2021 Jan 12;8:621644. doi: 10.3389/fcell.2020.621644. eCollection 2020. Front Cell Dev Biol. 2021. PMID: 33511134 Free PMC article. Review.
References
-
- Ingalls N.W. A human embryo at the beginning of segmentation, with special reference to the vascular system. Contr. Embryol. Carneg. Instn. 1920;11:61–90.
-
- Davis C.L. The development of the human heart from its first appearance to the stage found in embryos of twenty paired somites. Carnegie Inst. Wash. Publ. 380 Contr. Embryol. 1927;19:245–284.
-
- Streeter G.L. Developmental horizons in human embryos. Description of age group XIII, embryos about 4 or 5 millimeters long, and age group XIV, period of indentation of the lens vesicle. Carnegie Inst. Wash. Publ. 557 Contrib. Embryol. 1945;31:29–63.
Publication types
LinkOut - more resources
Full Text Sources
Miscellaneous