Novel Approaches for The Development of Live Attenuated Influenza Vaccines
- PMID: 30813325
- PMCID: PMC6409754
- DOI: 10.3390/v11020190
Novel Approaches for The Development of Live Attenuated Influenza Vaccines
Abstract
Influenza virus still represents a considerable threat to global public health, despite the advances in the development and wide use of influenza vaccines. Vaccination with traditional inactivate influenza vaccines (IIV) or live-attenuated influenza vaccines (LAIV) remains the main strategy in the control of annual seasonal epidemics, but it does not offer protection against new influenza viruses with pandemic potential, those that have shifted. Moreover, the continual antigenic drift of seasonal circulating influenza viruses, causing an antigenic mismatch that requires yearly reformulation of seasonal influenza vaccines, seriously compromises vaccine efficacy. Therefore, the quick optimization of vaccine production for seasonal influenza and the development of new vaccine approaches for pandemic viruses is still a challenge for the prevention of influenza infections. Moreover, recent reports have questioned the effectiveness of the current LAIV because of limited protection, mainly against the influenza A virus (IAV) component of the vaccine. Although the reasons for the poor protection efficacy of the LAIV have not yet been elucidated, researchers are encouraged to develop new vaccination approaches that overcome the limitations that are associated with the current LAIV. The discovery and implementation of plasmid-based reverse genetics has been a key advance in the rapid generation of recombinant attenuated influenza viruses that can be used for the development of new and most effective LAIV. In this review, we provide an update regarding the progress that has been made during the last five years in the development of new LAIV and the innovative ways that are being explored as alternatives to the currently licensed LAIV. The safety, immunogenicity, and protection efficacy profile of these new LAIVs reveal their possible implementation in combating influenza infections. However, efforts by vaccine companies and government agencies will be needed for controlled testing and approving, respectively, these new vaccine methodologies for the control of influenza infections.
Keywords: immunogenicity; influenza inactivated vaccine (IIV); influenza reverse genetics; influenza vaccines; influenza virus; live-attenuated influenza vaccine (LAIV); protection efficacy; recombinant influenza virus.
Conflict of interest statement
The authors declare no conflict of interest.
Figures
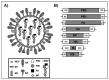
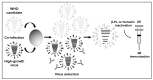
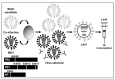
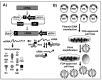
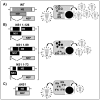
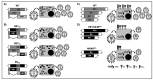
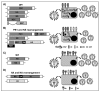
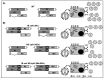
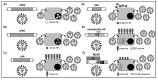
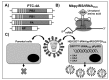
Similar articles
-
A Live Attenuated Influenza Vaccine Elicits Enhanced Heterologous Protection When the Internal Genes of the Vaccine Are Matched to Those of the Challenge Virus.J Virol. 2020 Jan 31;94(4):e01065-19. doi: 10.1128/JVI.01065-19. Print 2020 Jan 31. J Virol. 2020. PMID: 31748399 Free PMC article.
-
Generation of DelNS1 Influenza Viruses: a Strategy for Optimizing Live Attenuated Influenza Vaccines.mBio. 2019 Sep 17;10(5):e02180-19. doi: 10.1128/mBio.02180-19. mBio. 2019. PMID: 31530680 Free PMC article.
-
Rationale for vaccination with trivalent or quadrivalent live attenuated influenza vaccines: Protective vaccine efficacy in the ferret model.PLoS One. 2018 Dec 3;13(12):e0208028. doi: 10.1371/journal.pone.0208028. eCollection 2018. PLoS One. 2018. PMID: 30507951 Free PMC article.
-
Safety, immunogenicity and infectivity of new live attenuated influenza vaccines.Expert Rev Vaccines. 2015;14(10):1313-29. doi: 10.1586/14760584.2015.1075883. Epub 2015 Aug 6. Expert Rev Vaccines. 2015. PMID: 26289975 Review.
-
Immune responses after live attenuated influenza vaccination.Hum Vaccin Immunother. 2018 Mar 4;14(3):571-578. doi: 10.1080/21645515.2017.1377376. Epub 2018 Jan 3. Hum Vaccin Immunother. 2018. PMID: 28933664 Free PMC article. Review.
Cited by
-
Recombinant Influenza A Viruses Expressing Reporter Genes from the Viral NS Segment.Int J Mol Sci. 2024 Oct 1;25(19):10584. doi: 10.3390/ijms251910584. Int J Mol Sci. 2024. PMID: 39408912 Free PMC article. Review.
-
Ex Pluribus Unum: The CD4 T Cell Response against Influenza A Virus.Cells. 2024 Apr 5;13(7):639. doi: 10.3390/cells13070639. Cells. 2024. PMID: 38607077 Free PMC article. Review.
-
Antiviral responses versus virus-induced cellular shutoff: a game of thrones between influenza A virus NS1 and SARS-CoV-2 Nsp1.Front Cell Infect Microbiol. 2024 Feb 5;14:1357866. doi: 10.3389/fcimb.2024.1357866. eCollection 2024. Front Cell Infect Microbiol. 2024. PMID: 38375361 Free PMC article. Review.
-
Methionine enkephalin(MENK) upregulated memory T cells in anti-influenza response.BMC Immunol. 2023 Oct 12;24(1):38. doi: 10.1186/s12865-023-00573-0. BMC Immunol. 2023. PMID: 37828468 Free PMC article.
-
Nasal shedding of vaccine viruses after immunization with a Russian-backbone live attenuated influenza vaccine in India.Influenza Other Respir Viruses. 2023 Jun;17(6):e13149. doi: 10.1111/irv.13149. Influenza Other Respir Viruses. 2023. PMID: 37380175 Free PMC article. Clinical Trial.
References
-
- Shaw M.L., Palease P. Orthomyxoviridae: The viruses and their replication. In: Knipe D.M., Howley P.M., Griffin D.E., Lamb R.A., Martin M.A., editors. Fields Virology. 5th ed. Lippincott Williams and WIlkins; Philadelphia, PA, USA: 2007.
Publication types
MeSH terms
Substances
Grants and funding
LinkOut - more resources
Full Text Sources
Other Literature Sources
Medical