Rosiglitazone remodels the lipid droplet and britens human visceral and subcutaneous adipocytes ex vivo
- PMID: 30782959
- PMCID: PMC6446708
- DOI: 10.1194/jlr.M091173
Rosiglitazone remodels the lipid droplet and britens human visceral and subcutaneous adipocytes ex vivo
Abstract
Treatment with PPARγ agonists in vivo improves human adipocyte metabolism, but the cellular mechanisms and possible depot differences in responsiveness to their effects are poorly understood. To examine the ex vivo metabolic effects of rosiglitazone (Rosi), we cultured explants of human visceral (omental) and abdominal subcutaneous adipose tissues for 7 days. Rosi increased mRNA levels of transcriptional regulators of brite/beige adipocytes (PGC1α, PRDM16), triglyceride synthesis (GPAT3, DGAT1), and lipolysis (ATGL) similarly in adipose tissues from both depots. In parallel, Rosi increased key modulators of FA oxidation (UCP1, FABP3, PLIN5 protein), rates of FA oxidation, and protein levels of electron transport complexes, suggesting an enhanced respiratory capacity as confirmed in newly differentiated adipocytes. Rosi led to the formation of small lipid droplets (SLDs) around the adipocyte central lipid droplet; each SLD was decorated with redistributed mitochondria that colocalized with PLIN5. SLD maintenance required lipolysis and FA reesterification. Rosi thus coordinated a structural and metabolic remodeling in adipocytes from both visceral and subcutaneous depots that enhanced oxidative capacity. Selective targeting of these cellular mechanisms to improve adipocyte FA handling may provide a new approach to treat metabolic complications of obesity and diabetes.
Keywords: adipose depots; fatty acid oxidation; perilipins; protein carbonylation; thiazolidinedione.
Copyright © 2019 Lee et al.
Conflict of interest statement
The authors declare no competing financial interests.
Figures
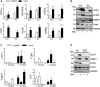
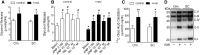
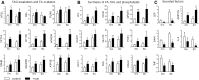
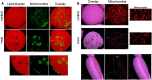
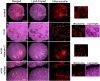
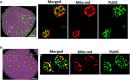
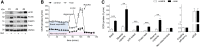

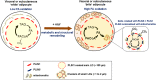
Similar articles
-
Metabolic and structural remodeling during browning of primary human adipocytes derived from omental and subcutaneous depots.Obesity (Silver Spring). 2024 Jan;32(1):70-79. doi: 10.1002/oby.23912. Epub 2023 Nov 6. Obesity (Silver Spring). 2024. PMID: 37929774
-
Adipocyte-specific Hypoxia-inducible gene 2 promotes fat deposition and diet-induced insulin resistance.Mol Metab. 2016 Sep 28;5(12):1149-1161. doi: 10.1016/j.molmet.2016.09.009. eCollection 2016 Dec. Mol Metab. 2016. PMID: 27900258 Free PMC article.
-
Combined effects of rosiglitazone and conjugated linoleic acid on adiposity, insulin sensitivity, and hepatic steatosis in high-fat-fed mice.Am J Physiol Gastrointest Liver Physiol. 2007 Jun;292(6):G1671-82. doi: 10.1152/ajpgi.00523.2006. Epub 2007 Feb 22. Am J Physiol Gastrointest Liver Physiol. 2007. PMID: 17322064
-
Triphenyl phosphate is a selective PPARγ modulator that does not induce brite adipogenesis in vitro and in vivo.Arch Toxicol. 2020 Sep;94(9):3087-3103. doi: 10.1007/s00204-020-02815-1. Epub 2020 Jul 18. Arch Toxicol. 2020. PMID: 32683515 Free PMC article.
-
Role of energy charge and AMP-activated protein kinase in adipocytes in the control of body fat stores.Int J Obes Relat Metab Disord. 2004 Dec;28 Suppl 4:S38-44. doi: 10.1038/sj.ijo.0802855. Int J Obes Relat Metab Disord. 2004. PMID: 15592485 Review.
Cited by
-
Remodeling of Adipose Tissues by Fatty Acids: Mechanistic Update on Browning and Thermogenesis by n-3 Polyunsaturated Fatty Acids.Pharm Res. 2023 Feb;40(2):467-480. doi: 10.1007/s11095-022-03377-w. Epub 2022 Sep 1. Pharm Res. 2023. PMID: 36050546 Review.
-
The biology of lipid droplet-bound mitochondria.Semin Cell Dev Biol. 2020 Dec;108:55-64. doi: 10.1016/j.semcdb.2020.04.013. Epub 2020 May 20. Semin Cell Dev Biol. 2020. PMID: 32446655 Free PMC article. Review.
-
IRF8 and its related molecules as potential diagnostic biomarkers or therapeutic candidates and immune cell infiltration characteristics in steroid-induced osteonecrosis of the femoral head.J Orthop Surg Res. 2023 Jan 10;18(1):27. doi: 10.1186/s13018-022-03381-1. J Orthop Surg Res. 2023. PMID: 36627660 Free PMC article.
-
Preventing White Adipocyte Browning during Differentiation In Vitro: The Effect of Differentiation Protocols on Metabolic and Mitochondrial Phenotypes.Stem Cells Int. 2022 Apr 5;2022:3308194. doi: 10.1155/2022/3308194. eCollection 2022. Stem Cells Int. 2022. PMID: 35422865 Free PMC article.
-
Essential Roles of PPARs in Lipid Metabolism during Mycobacterial Infection.Int J Mol Sci. 2021 Jul 15;22(14):7597. doi: 10.3390/ijms22147597. Int J Mol Sci. 2021. PMID: 34299217 Free PMC article. Review.
References
-
- Gustafson B., Hedjazifar S., Gogg S., Hammarstedt A., and Smith U.. 2015. Insulin resistance and impaired adipogenesis. Trends Endocrinol. Metab. 26: 193–200. - PubMed
-
- Koutsari C., and Jensen M. D.. 2006. Free fatty acid metabolism in human obesity. J. Lipid Res. 47: 1643–1650. - PubMed
-
- Das S. K., Chu W. S., Mondal A. K., Sharma N. K., Kern P. A., Rasouli N., and Elbein S. C.. 2008. Effect of pioglitazone treatment on endoplasmic reticulum stress response in human adipose and in palmitate-induced stress in human liver and adipose cell lines. Am. J. Physiol. Endocrinol. Metab. 295: E393–E400. - PMC - PubMed
-
- Frayn K. N., Langin D., and Karpe F.. 2008. Fatty acid-induced mitochondrial uncoupling in adipocytes is not a promising target for treatment of insulin resistance unless adipocyte oxidative capacity is increased. Diabetologia. 51: 394–397. - PubMed
Publication types
MeSH terms
Substances
Grants and funding
LinkOut - more resources
Full Text Sources
Medical
Molecular Biology Databases
Miscellaneous