Unraveling the mechanisms of calcium-dependent secretion
- PMID: 30782604
- PMCID: PMC6445591
- DOI: 10.1085/jgp.201812298
Unraveling the mechanisms of calcium-dependent secretion
Abstract
Ca2+-dependent secretion is a process by which important signaling molecules that are produced within a cell-including proteins and neurotransmitters-are expelled to the extracellular environment. The cellular mechanism that underlies secretion is referred to as exocytosis. Many years of work have revealed that exocytosis in neurons and neuroendocrine cells is tightly coupled to Ca2+ and orchestrated by a series of protein-protein/protein-lipid interactions. Here, we highlight landmark discoveries that have informed our current understanding of the process. We focus principally on reductionist studies performed using powerful model secretory systems and cell-free reconstitution assays. In recent years, molecular cloning and genetics have implicated the involvement of a sizeable number of proteins in exocytosis. We expect reductionist approaches will be central to attempts to resolve their roles. The Journal of General Physiology will continue to be an outlet for much of this work, befitting its tradition of publishing strongly mechanistic, basic research.
© 2019 Anantharam and Kreutzberger.
Figures
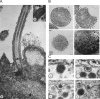
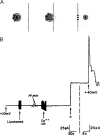
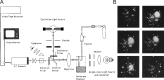
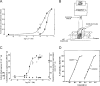
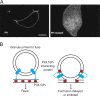
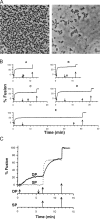
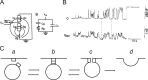
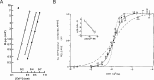
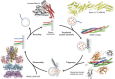
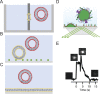
Similar articles
-
Temporal and spatial coordination of exocytosis and endocytosis.Nat Rev Mol Cell Biol. 2003 Feb;4(2):127-39. doi: 10.1038/nrm1016. Nat Rev Mol Cell Biol. 2003. PMID: 12563290 Review.
-
High calcium concentrations shift the mode of exocytosis to the kiss-and-run mechanism.Nat Cell Biol. 1999 May;1(1):40-4. doi: 10.1038/9012. Nat Cell Biol. 1999. PMID: 10559862
-
Secretory carrier membrane protein SCAMP2 and phosphatidylinositol 4,5-bisphosphate interactions in the regulation of dense core vesicle exocytosis.Biochemistry. 2007 Sep 25;46(38):10909-20. doi: 10.1021/bi701121j. Epub 2007 Aug 22. Biochemistry. 2007. PMID: 17713930
-
An activity-dependent increased role for L-type calcium channels in exocytosis is regulated by adrenergic signaling in chromaffin cells.Neuroscience. 2006 Dec 1;143(2):445-59. doi: 10.1016/j.neuroscience.2006.08.001. Epub 2006 Sep 8. Neuroscience. 2006. PMID: 16962713
-
[Bacterial toxins: useful for studying G-proteins implicated in the mechanism of exocytosis in neuroendocrine cells].J Soc Biol. 1999;193(6):451-6. J Soc Biol. 1999. PMID: 10783703 Review. French.
Cited by
-
Mitochondrial Bioenergetics and Dynamics in Secretion Processes.Front Endocrinol (Lausanne). 2020 May 22;11:319. doi: 10.3389/fendo.2020.00319. eCollection 2020. Front Endocrinol (Lausanne). 2020. PMID: 32528413 Free PMC article. Review.
-
Secretory MPP3 reinforce myeloid differentiation trajectory and amplify myeloid cell production.J Exp Med. 2023 Aug 7;220(8):e20230088. doi: 10.1084/jem.20230088. Epub 2023 Apr 26. J Exp Med. 2023. PMID: 37115584 Free PMC article.
-
Nav1.7 as a chondrocyte regulator and therapeutic target for osteoarthritis.Nature. 2024 Jan;625(7995):557-565. doi: 10.1038/s41586-023-06888-7. Epub 2024 Jan 3. Nature. 2024. PMID: 38172636 Free PMC article.
-
In silico study on identification of novel MALT1 allosteric inhibitors.RSC Adv. 2019 Nov 29;9(67):39338-39347. doi: 10.1039/c9ra07036b. eCollection 2019 Nov 27. RSC Adv. 2019. PMID: 35540679 Free PMC article.
-
PACAP and acetylcholine cause distinct Ca2+ signals and secretory responses in chromaffin cells.J Gen Physiol. 2023 Feb 6;155(2):e202213180. doi: 10.1085/jgp.202213180. Epub 2022 Dec 20. J Gen Physiol. 2023. PMID: 36538657 Free PMC article.
References
Publication types
MeSH terms
Substances
Grants and funding
LinkOut - more resources
Full Text Sources
Miscellaneous