Tetrazoles via Multicomponent Reactions
- PMID: 30707567
- PMCID: PMC6376451
- DOI: 10.1021/acs.chemrev.8b00564
Tetrazoles via Multicomponent Reactions
Abstract
Tetrazole derivatives are a prime class of heterocycles, very important to medicinal chemistry and drug design due to not only their bioisosterism to carboxylic acid and amide moieties but also to their metabolic stability and other beneficial physicochemical properties. Although more than 20 FDA-approved drugs contain 1 H- or 2 H-tetrazole substituents, their exact binding mode, structural biology, 3D conformations, and in general their chemical behavior is not fully understood. Importantly, multicomponent reaction (MCR) chemistry offers convergent access to multiple tetrazole scaffolds providing the three important elements of novelty, diversity, and complexity, yet MCR pathways to tetrazoles are far from completely explored. Here, we review the use of multicomponent reactions for the preparation of substituted tetrazole derivatives. We highlight specific applications and general trends holding therein and discuss synthetic approaches and their value by analyzing scope and limitations, and also enlighten their receptor binding mode. Finally, we estimated the prospects of further research in this field.
Conflict of interest statement
The authors declare no competing financial interest.
Figures
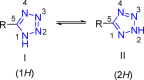
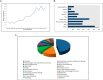
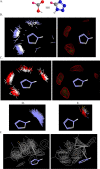
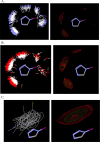
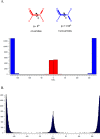
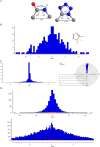
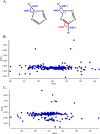
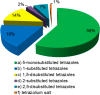
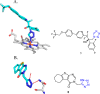
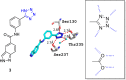
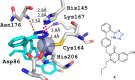
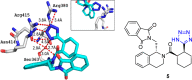
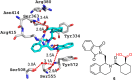
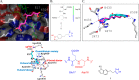
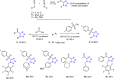
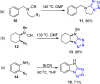
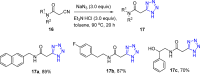
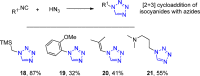
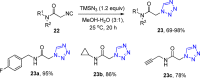
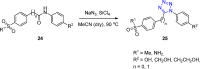
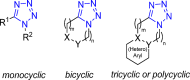
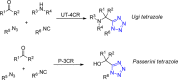
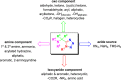
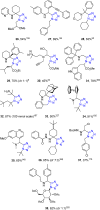
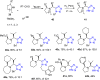
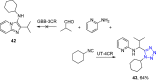
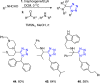
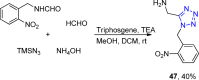
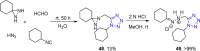
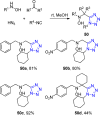

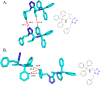
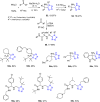
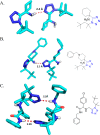
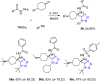
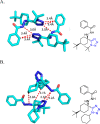
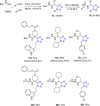
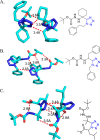
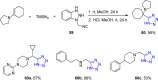

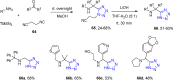
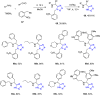
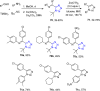
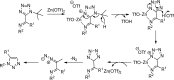
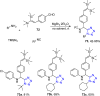
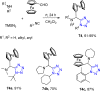
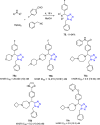
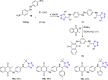
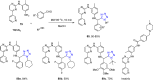
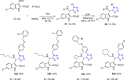
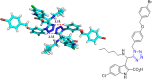
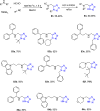
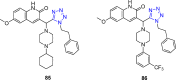
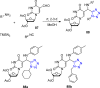
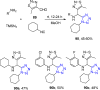
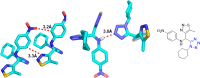
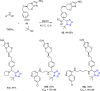
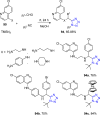
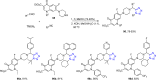
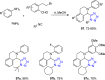
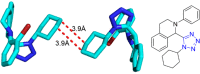
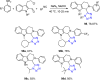
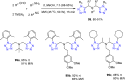
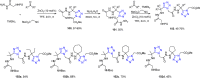
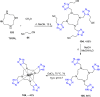
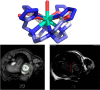
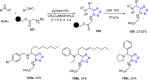
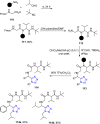
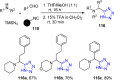
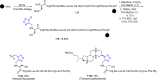
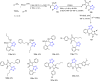
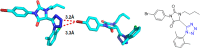
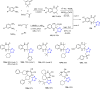
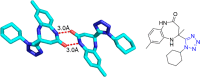
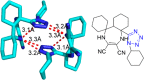
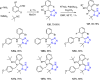
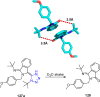
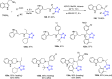
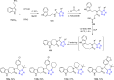
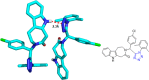
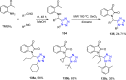
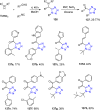
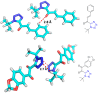
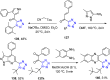
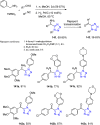
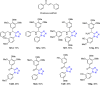
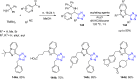
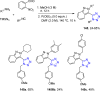
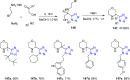
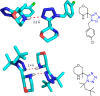
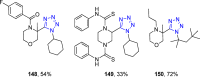
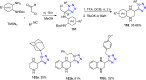
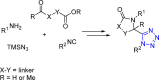
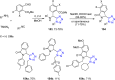
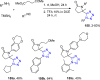
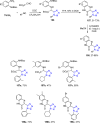
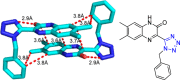
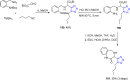
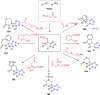
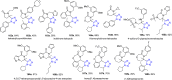

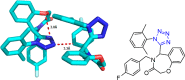
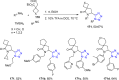
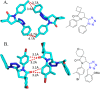
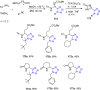
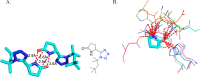
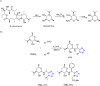
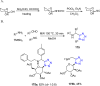
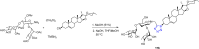
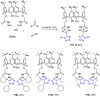
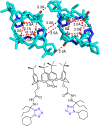
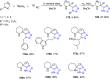
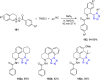
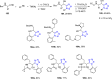
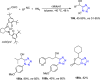
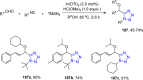
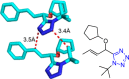
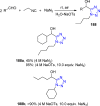
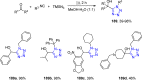
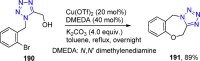
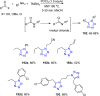
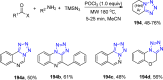
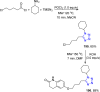

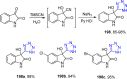
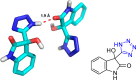
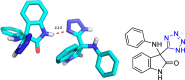
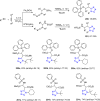
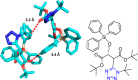
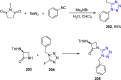
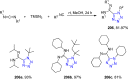
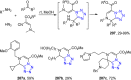
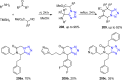
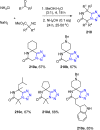
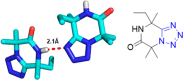
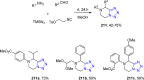
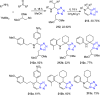
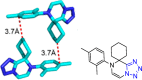
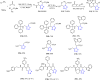
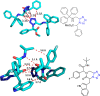
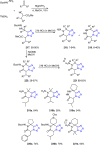
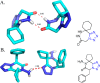
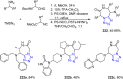
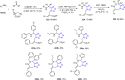
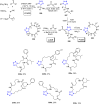
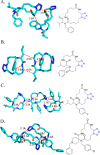
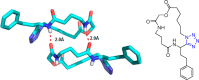
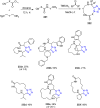
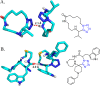
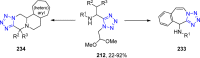
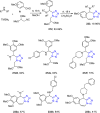
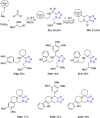
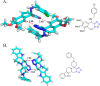
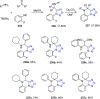
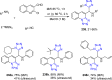
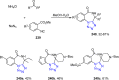
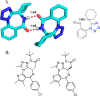
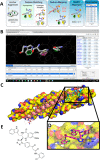
Similar articles
-
Tetrazoles: A multi-potent motif in drug design.Eur J Med Chem. 2024 Dec 5;279:116870. doi: 10.1016/j.ejmech.2024.116870. Epub 2024 Sep 14. Eur J Med Chem. 2024. PMID: 39316842 Review.
-
α-Amino Acid-Isosteric α-Amino Tetrazoles.Chemistry. 2016 Feb 24;22(9):3009-18. doi: 10.1002/chem.201504520. Epub 2016 Jan 28. Chemistry. 2016. PMID: 26817531 Free PMC article.
-
The hydrogen bond environments of 1H-tetrazole and tetrazolate rings: the structural basis for tetrazole-carboxylic acid bioisosterism.J Chem Inf Model. 2012 Mar 26;52(3):857-66. doi: 10.1021/ci200521k. Epub 2012 Feb 21. J Chem Inf Model. 2012. PMID: 22303876
-
5-Substituted-1H-tetrazoles as carboxylic acid isosteres: medicinal chemistry and synthetic methods.Bioorg Med Chem. 2002 Nov;10(11):3379-93. doi: 10.1016/s0968-0896(02)00239-0. Bioorg Med Chem. 2002. PMID: 12213451 Review.
-
Tetrazole Derivatives as Promising Anticancer Agents.Anticancer Agents Med Chem. 2018 Feb 7;17(14):1856-1868. doi: 10.2174/1871520617666170327143148. Anticancer Agents Med Chem. 2018. PMID: 28356016 Review.
Cited by
-
Nanomagnetic tetraaza (N4 donor) macrocyclic Schiff base complex of copper(ii): synthesis, characterizations, and its catalytic application in Click reactions.Nanoscale Adv. 2023 Oct 20;5(23):6594-6605. doi: 10.1039/d3na00580a. eCollection 2023 Nov 21. Nanoscale Adv. 2023. PMID: 38024320 Free PMC article.
-
Cu(ii) immobilized on Fe3O4@HNTs-tetrazole (CFHT) nanocomposite: synthesis, characterization, investigation of its catalytic role for the 1,3 dipolar cycloaddition reaction, and antibacterial activity.RSC Adv. 2020 Jul 15;10(44):26467-26478. doi: 10.1039/d0ra04772d. eCollection 2020 Jul 9. RSC Adv. 2020. PMID: 35519782 Free PMC article.
-
Discovery of Dihydropyrrol-2-ones as Novel G0/G1-Phase Arresting Agents Inducing Apoptosis.ACS Omega. 2019 Oct 14;4(17):17556-17560. doi: 10.1021/acsomega.9b02543. eCollection 2019 Oct 22. ACS Omega. 2019. PMID: 31656929 Free PMC article.
-
Chloromethyl Glycosides as Versatile Synthons to Prepare Glycosyloxymethyl-Prodrugs.Chemistry. 2022 Feb 16;28(9):e202103910. doi: 10.1002/chem.202103910. Epub 2022 Jan 24. Chemistry. 2022. PMID: 35045197 Free PMC article.
-
Design, synthesis and biological evaluation of 1,5-disubstituted α-amino tetrazole derivatives as non-covalent inflammasome-caspase-1 complex inhibitors with potential application against immune and inflammatory disorders.Eur J Med Chem. 2022 Feb 5;229:114002. doi: 10.1016/j.ejmech.2021.114002. Epub 2021 Nov 18. Eur J Med Chem. 2022. PMID: 34823899 Free PMC article.
References
-
- Bhatt U.Five-Membered Heterocycles with Four Heteroatoms: Tetrazoles. In Modern Heterocyclic Chemistry; Wiley-VCH Verlag GmbH & Co. KGaA, 2011; pp 1401–1430.
-
- Bladin J. A. Ueber von Dicyanphenylhydrazin Abgeleitete Verbindungen. Ber. Dtsch. Chem. Ges. 1885, 18, 1544–1551. 10.1002/cber.188501801335. - DOI
Publication types
MeSH terms
Substances
Grants and funding
LinkOut - more resources
Full Text Sources