TFAP2C- and p63-Dependent Networks Sequentially Rearrange Chromatin Landscapes to Drive Human Epidermal Lineage Commitment
- PMID: 30686763
- PMCID: PMC7135956
- DOI: 10.1016/j.stem.2018.12.012
TFAP2C- and p63-Dependent Networks Sequentially Rearrange Chromatin Landscapes to Drive Human Epidermal Lineage Commitment
Abstract
Tissue development results from lineage-specific transcription factors (TFs) programming a dynamic chromatin landscape through progressive cell fate transitions. Here, we define epigenomic landscape during epidermal differentiation of human pluripotent stem cells (PSCs) and create inference networks that integrate gene expression, chromatin accessibility, and TF binding to define regulatory mechanisms during keratinocyte specification. We found two critical chromatin networks during surface ectoderm initiation and keratinocyte maturation, which are driven by TFAP2C and p63, respectively. Consistently, TFAP2C, but not p63, is sufficient to initiate surface ectoderm differentiation, and TFAP2C-initiated progenitor cells are capable of maturing into functional keratinocytes. Mechanistically, TFAP2C primes the surface ectoderm chromatin landscape and induces p63 expression and binding sites, thus allowing maturation factor p63 to positively autoregulate its own expression and close a subset of the TFAP2C-initiated surface ectoderm program. Our work provides a general framework to infer TF networks controlling chromatin transitions that will facilitate future regenerative medicine advances.
Copyright © 2018. Published by Elsevier Inc.
Figures
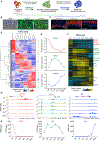
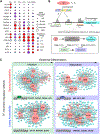
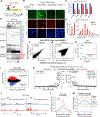
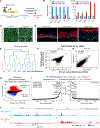
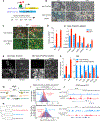
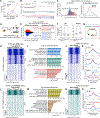
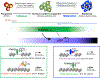
Similar articles
-
A novel ATAC-seq approach reveals lineage-specific reinforcement of the open chromatin landscape via cooperation between BAF and p63.Genome Biol. 2015 Dec 18;16:284. doi: 10.1186/s13059-015-0840-9. Genome Biol. 2015. PMID: 26683334 Free PMC article.
-
Notch signaling represses p63 expression in the developing surface ectoderm.Development. 2013 Sep;140(18):3777-86. doi: 10.1242/dev.093948. Epub 2013 Aug 7. Development. 2013. PMID: 23924630 Free PMC article.
-
Retinoic acid and BMP4 cooperate with p63 to alter chromatin dynamics during surface epithelial commitment.Nat Genet. 2018 Dec;50(12):1658-1665. doi: 10.1038/s41588-018-0263-0. Epub 2018 Nov 5. Nat Genet. 2018. PMID: 30397335 Free PMC article.
-
Master regulatory role of p63 in epidermal development and disease.Cell Mol Life Sci. 2018 Apr;75(7):1179-1190. doi: 10.1007/s00018-017-2701-z. Epub 2017 Nov 4. Cell Mol Life Sci. 2018. PMID: 29103147 Free PMC article. Review.
-
Dynamic life of a skin keratinocyte: an intimate tryst with the master regulator p63.Indian J Exp Biol. 2011 Oct;49(10):721-31. Indian J Exp Biol. 2011. PMID: 22013738 Review.
Cited by
-
Statistical learning quantifies transposable element-mediated cis-regulation.Genome Biol. 2023 Nov 10;24(1):258. doi: 10.1186/s13059-023-03085-7. Genome Biol. 2023. PMID: 37950299 Free PMC article.
-
AP-2α and AP-2β cooperatively function in the craniofacial surface ectoderm to regulate chromatin and gene expression dynamics during facial development.Elife. 2022 Mar 25;11:e70511. doi: 10.7554/eLife.70511. Elife. 2022. PMID: 35333176 Free PMC article.
-
Epigenetic and transcriptional regulations prime cell fate before division during human pluripotent stem cell differentiation.Nat Commun. 2023 Jan 25;14(1):405. doi: 10.1038/s41467-023-36116-9. Nat Commun. 2023. PMID: 36697417 Free PMC article.
-
Cis-regulatory chromatin loops analysis identifies GRHL3 as a master regulator of surface epithelium commitment.Sci Adv. 2022 Jul 15;8(28):eabo5668. doi: 10.1126/sciadv.abo5668. Epub 2022 Jul 13. Sci Adv. 2022. PMID: 35857527 Free PMC article.
-
Specific chromatin landscapes and transcription factors couple breast cancer subtype with metastatic relapse to lung or brain.BMC Med Genomics. 2020 Mar 6;13(1):33. doi: 10.1186/s12920-020-0695-0. BMC Med Genomics. 2020. PMID: 32143622 Free PMC article.
References
-
- Antonini D, Sirico A, Aberdam E, Ambrosio R, Campanile C, Fagoonee S, Altruda F, Aberdam D, Brissette JL, and Missero C. (2015). A composite enhancer regulates p63 gene expression in epidermal morphogenesis and in keratinocyte differentiation by multiple mechanisms. Nucleic Acids Res. 43, 862–874. - PMC - PubMed
-
- Auman HJ, Nottoli T, Lakiza O, Winger Q, Donaldson S, and Williams T. (2002). Transcription factor AP-2gamma is essential in the extra-embryonic lineages for early postimplantation development. Development 129, 2733–2747. - PubMed
-
- Bragança J, Eloranta JJ, Bamforth SD, Ibbitt JC, Hurst HC, and Bhattacharya S. (2003). Physical and functional interactions among AP-2 transcription factors, p300/CREB-binding protein, and CITED2. J. Biol. Chem. 278, 16021–16029. - PubMed
Publication types
MeSH terms
Substances
Grants and funding
LinkOut - more resources
Full Text Sources
Molecular Biology Databases
Research Materials
Miscellaneous