Shape-Persistent Actuators from Hydrazone Photoswitches
- PMID: 30624915
- PMCID: PMC6346373
- DOI: 10.1021/jacs.8b11558
Shape-Persistent Actuators from Hydrazone Photoswitches
Abstract
Interfacing molecular photoswitches with liquid crystal polymers enables the amplification of their nanoscale motion into macroscopic shape transformations. Typically, the mechanism responsible for actuation involves light-induced molecular disorder. Here, we demonstrate that bistable hydrazones can drive (chiral) shape transformations in liquid crystal polymer networks, with photogenerated polymer shapes displaying a long-term stability that mirrors that of the switches. The mechanism involves a photoinduced buildup of tension in the polymer, with a negligible influence on the liquid crystalline order. Hydrazone-doped liquid crystal systems thus diversify the toolbox available to the field of light-adaptive molecular actuators and hold promise in terms of soft robotics.
Conflict of interest statement
The authors declare no competing financial interest.
Figures
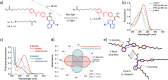
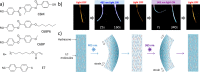
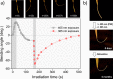
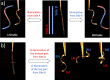
Similar articles
-
Fluorinated Azobenzenes for Shape-Persistent Liquid Crystal Polymer Networks.Angew Chem Int Ed Engl. 2016 Aug 16;55(34):9908-12. doi: 10.1002/anie.201603579. Epub 2016 Jul 19. Angew Chem Int Ed Engl. 2016. PMID: 27430357
-
Self-sustained actuation from heat dissipation in liquid crystal polymer networks.J Polym Sci A Polym Chem. 2018 Jul 1;56(13):1331-1336. doi: 10.1002/pola.29032. Epub 2018 Apr 27. J Polym Sci A Polym Chem. 2018. PMID: 29937627 Free PMC article.
-
Hydrazone Photoswitches for Structural Modulation of Short Peptides.Chemistry. 2022 Feb 19;28(11):e202103972. doi: 10.1002/chem.202103972. Epub 2022 Jan 28. Chemistry. 2022. PMID: 34962683
-
Liquid crystal polymer actuators with complex and multiple actuations.J Mater Chem B. 2024 Jul 17;12(28):6757-6773. doi: 10.1039/d4tb01055h. J Mater Chem B. 2024. PMID: 38916076 Review.
-
Electrically driven liquid crystal network actuators.Soft Matter. 2022 Jul 6;18(26):4850-4867. doi: 10.1039/d2sm00544a. Soft Matter. 2022. PMID: 35730498 Review.
Cited by
-
Thermally Stable Photomechanical Molecular Hinge: Sterically Hindered Stiff-Stilbene Photoswitch Mechanically Isomerizes.JACS Au. 2023 Aug 21;3(9):2458-2466. doi: 10.1021/jacsau.3c00213. eCollection 2023 Sep 25. JACS Au. 2023. PMID: 37772185 Free PMC article.
-
An artificial aquatic polyp that wirelessly attracts, grasps, and releases objects.Proc Natl Acad Sci U S A. 2020 Jul 28;117(30):17571-17577. doi: 10.1073/pnas.2004748117. Epub 2020 Jul 13. Proc Natl Acad Sci U S A. 2020. PMID: 32661153 Free PMC article.
-
Arene Substitution Design for Controlled Conformational Changes of Dibenzocycloocta-1,5-dienes.J Am Chem Soc. 2020 Sep 30;142(39):16651-16660. doi: 10.1021/jacs.0c06579. Epub 2020 Sep 17. J Am Chem Soc. 2020. PMID: 32881488 Free PMC article.
-
Antidiabetic Wound Dressing Materials Based on Cellulosic Fabrics Loaded with Zinc Oxide Nanoparticles Synthesized by Solid-State Method.Polymers (Basel). 2022 May 27;14(11):2168. doi: 10.3390/polym14112168. Polymers (Basel). 2022. PMID: 35683840 Free PMC article.
-
Directional, Low-Energy Driven Thermal Actuating Bilayer Enabled by Coordinated Submolecular Switching.Adv Sci (Weinh). 2021 Dec;8(23):e2102077. doi: 10.1002/advs.202102077. Epub 2021 Oct 23. Adv Sci (Weinh). 2021. PMID: 34687166 Free PMC article.
References
-
- Browne W. R.; Feringa B. L. Making Molecular Machines Work. Nat. Nanotechnol. 2006, 1, 25–35. 10.1038/nnano.2006.45. - DOI - PubMed
- Coskun A.; Banaszak M.; Astumian R. D.; Stoddart J. F.; Grzybowski B. A. Great Expectations: Can Artificial Molecular Machines Deliver on their Promise?. Chem. Soc. Rev. 2012, 41, 19–31. 10.1039/C1CS15262A. - DOI - PubMed
- Zhang L.; Marcos V.; Leigh D. A. Molecular Machines with Bio-inspired Mechanisms. Proc. Natl. Acad. Sci. U. S. A. 2018, 115, 9397–9404. 10.1073/pnas.1712788115. - DOI - PMC - PubMed
- Abendroth J. M.; Bushuyev O. S.; Weiss P. S.; Barrett C. J. Controlling Motion at the Nanoscale: Rise of the Molecular Machines. ACS Nano 2015, 9, 7746–7768. 10.1021/acsnano.5b03367. - DOI - PubMed
- Ornes S. What’s the best way to build a molecular machine?. Proc. Natl. Acad. Sci. U. S. A. 2018, 115, 9327–9330. 10.1073/pnas.1811689115. - DOI - PMC - PubMed
-
- Eelkema R.; Pollard M. M.; Vicario J.; Katsonis N.; Ramon B. S.; Bastiaansen C. W.; Broer D. J.; Feringa B. L. Molecular Machines: Nanomotor Rotates Microscale Objects. Nature 2006, 440, 163.10.1038/440163a. - DOI - PubMed
- Chen J.; Leung F. K-C.; Stuart M. C. A.; Kajitani T.; Fukushima T.; van der Giessen E.; Feringa B. L. Artificial Muscle-Like Function From Hierarchical Supramolecular Assembly of Photoresponsive Molecular Motors. Nat. Chem. 2017, 10, 132–138. 10.1038/nchem.2887. - DOI - PubMed
-
- Klajn R.; Bléger D. Integrating Macromolecules with Molecular Switches. Macromol. Rapid Commun. 2018, 39, 1700827.10.1002/marc.201700827. - DOI - PubMed
- Fredy J. W.; Méndez-Ardoy A.; Kwangmettatam S.; Bochicchio D.; Matt B.; Stuart M. C. A.; Huskens J.; Katsonis N.; Pavan G. M.; Kudernac T. Molecular Photoswitches Mediating the Strain-Driven Disassembly of Supramolecular Tubules. Proc. Natl. Acad. Sci. U. S. A. 2017, 114, 11850–11855. 10.1073/pnas.1711184114. - DOI - PMC - PubMed
-
- Morone M. I. Harnessing the Power of Shape-Shifting Polymers. Chem. Eng. News 2018, 96, 36.
- Davenport M. Packing More Punch Into Polymer Devices. Chem. Eng. News 2017, 95, 11.
-
- Fischer P.; Palagi S. Bioinspired Microrobots. Nat. Rev. Mater. 2018, 3, 113–124. 10.1038/s41578-018-0016-9. - DOI
Publication types
LinkOut - more resources
Full Text Sources
Other Literature Sources