Glucose starvation triggers filamentous septin assemblies in an S. pombe septin-2 deletion mutant
- PMID: 30602528
- PMCID: PMC6361201
- DOI: 10.1242/bio.037622
Glucose starvation triggers filamentous septin assemblies in an S. pombe septin-2 deletion mutant
Abstract
Using correlative light and electron microscopy (CLEM), we studied the intracellular organization by of glucose-starved fission yeast cells (Schizosaccharomyces pombe) with regards to the localization of septin proteins throughout the cytoplasm. Thereby, we found that for cells carrying a deletion of the gene encoding septin-2 (spn2Δ), starvation causes a GFP-tagged version of septin-3 (spn3-GFP) and family members, to assemble into a single, prominent filamentous structure. It was previously shown that during exponential growth, spn2Δ cells form septin-3 polymers. However, the polymers we observed during exponential growth are different from the spn3p-GFP structure we observed in starved cells. Using CLEM, in combination with anti-GFP immunolabeling on plastic-sections, we could assign spn3p-GFP to the filaments we have found in EM pictures. Besides septin-3, these filamentous assemblies most likely also contain septin-1 as an RFP-tagged version of this protein forms a very similar structure in starved spn2Δ cells. Our data correlate phase-contrast and fluorescence microscopy with electron micrographs of plastic-embedded cells, and further on with detailed views of tomographic 3D reconstructions. Cryo-electron microscopy of spn2Δ cells in vitrified sections revealed a very distinct overall morphology of the spn3p-GFP assembly. The fine-structured, regular density pattern suggests the presence of assembled septin-3 filaments that are clearly different from F-actin bundles. Furthermore, we found that starvation causes substantial mitochondria fission, together with massive decoration of their outer membrane by ribosomes.
Keywords: Conventional and cryo-electron microscopy; Correlative light and electron microscopy; Filamentous septin assemblies; Glucose starvation in S. pombe; Septins; Vitrified sectioning.
© 2019. Published by The Company of Biologists Ltd.
Conflict of interest statement
Competing interestsThe authors declare no competing or financial interests.
Figures
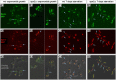
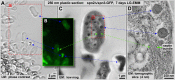
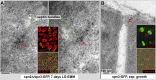
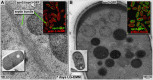
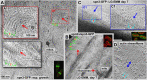
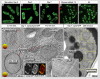
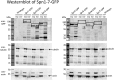
Similar articles
-
Changes in the distribution of F-actin in the fission yeast Schizosaccharomyces pombe by arresting growth in distilled water: correlative studies with fluorescence and electron microscopy.J Electron Microsc (Tokyo). 1994 Feb;43(1):20-4. J Electron Microsc (Tokyo). 1994. PMID: 8021562
-
Ashbya gossypii as a model system to study septin organization by single-molecule localization microscopy.Methods Cell Biol. 2016;136:161-82. doi: 10.1016/bs.mcb.2016.04.007. Epub 2016 Jun 1. Methods Cell Biol. 2016. PMID: 27473909
-
Characterization of the mammalian septin H5: distinct patterns of cytoskeletal and membrane association from other septin proteins.Cell Motil Cytoskeleton. 1999;43(1):52-62. doi: 10.1002/(SICI)1097-0169(1999)43:1<52::AID-CM6>3.0.CO;2-5. Cell Motil Cytoskeleton. 1999. PMID: 10340703
-
Protein-protein interactions governing septin heteropentamer assembly and septin filament organization in Saccharomyces cerevisiae.Mol Biol Cell. 2004 Oct;15(10):4568-83. doi: 10.1091/mbc.e04-04-0330. Epub 2004 Jul 28. Mol Biol Cell. 2004. PMID: 15282341 Free PMC article.
-
Guides to the final frontier of the cytoskeleton: septins in filamentous fungi.Curr Opin Microbiol. 2010 Dec;13(6):720-6. doi: 10.1016/j.mib.2010.09.012. Epub 2010 Oct 9. Curr Opin Microbiol. 2010. PMID: 20934902 Review.
Cited by
-
The Roles of Septins in Regulating Fission Yeast Cytokinesis.J Fungi (Basel). 2024 Jan 30;10(2):115. doi: 10.3390/jof10020115. J Fungi (Basel). 2024. PMID: 38392788 Free PMC article. Review.
-
Neuronal-specific septin-3 binds Atg8/LC3B, accumulates and localizes to autophagosomes during induced autophagy.Cell Mol Life Sci. 2022 Aug 6;79(9):471. doi: 10.1007/s00018-022-04488-8. Cell Mol Life Sci. 2022. PMID: 35932293 Free PMC article.
-
Ribosomes hibernate on mitochondria during cellular stress.Nat Commun. 2024 Oct 8;15(1):8666. doi: 10.1038/s41467-024-52911-4. Nat Commun. 2024. PMID: 39379376 Free PMC article.
References
-
- Bähler J., Wu J.-Q., Longtine M. S., Shah N. G., McKenzie A. III, Steever A. B., Wach A., Philippsen P. and Pringle J. R. (1998). Heterologous modules for efficient and versatile PCR-based gene targeting in Schizosaccharomyces pombe. Yeast 14, 943-951. 10.1002/(SICI)1097-0061(199807)14:10<943::AID-YEA292>3.0.CO;2-Y - DOI - PubMed
Grants and funding
LinkOut - more resources
Full Text Sources
Research Materials